Product Manager
- FMA
- The Fabricator
- FABTECH
- Canadian Metalworking
Categories
- Additive Manufacturing
- Aluminum Welding
- Arc Welding
- Assembly and Joining
- Automation and Robotics
- Bending and Forming
- Consumables
- Cutting and Weld Prep
- Electric Vehicles
- En Español
- Finishing
- Hydroforming
- Laser Cutting
- Laser Welding
- Machining
- Manufacturing Software
- Materials Handling
- Metals/Materials
- Oxyfuel Cutting
- Plasma Cutting
- Power Tools
- Punching and Other Holemaking
- Roll Forming
- Safety
- Sawing
- Shearing
- Shop Management
- Testing and Measuring
- Tube and Pipe Fabrication
- Tube and Pipe Production
- Waterjet Cutting
Industry Directory
Webcasts
Podcasts
FAB 40
Advertise
Subscribe
Account Login
Search
Strong metal, light cutting
Multiaxis laser cutting opens the door for hot-formed steel
- By Frank Geyer
- March 7, 2013
- Article
- Laser Cutting
The automotive world is undergoing a sea change in vehicle design. Fuel efficiency and crash protection requirements have led to smaller and lighter yet stronger passenger vehicles. Center stage in this development is ultrahigh-strength steel (UHSS) 22MnB5—or, simply, hot-formed steel.
As just one example, hot-formed steel now makes up the structural sheet metal parts for vehicle passenger compartments. The A- and B- pillars, for instance, are well-suited for this application, in which hot-formed steel helps reduce metal deformation during a collision, protecting the passengers inside.
Hot forming starts with blanking raw parts from coiled material, an AlSi-coated boron steel with an initial yield strength of 500 MPa. The blanks then are heated to approximately 1,200 degrees F to ensure the AlSi coating bonds to the boron steel base. Next, they are further heated to approximately 1,740 degrees F, formed and then immediately quenched while still hot and in the press. Within 25 seconds the parts cool to 400 degrees F, when the material structure becomes austenitic, with a yield strength of around 1,500 MPa.
After the blanks are formed, typical production steps include cutting holes or openings and trimming. But the material’s extremely high strength makes cutting and trimming with hard dies impractical, even infeasible. This is where the multiaxis laser finds its niche. With its focused, high energy density, the laser melts the hot-formed steel and allows the high-pressure cutting gas (nitrogen or compressed air) to expel the molten mass out of the cut. This produces a smooth cutting edge with only a small heat-affected zone.
The challenge, however, lies in matching the throughput of laser cutting with that of blanking. For any exterior trim or interior cutout, a laser cuts one contour after another, while a die cuts all at once.
Cutting Speed Improvements
Cutting speed has improved considerably over the years. Consider processing improvements for the Volkswagen Passat® B- pillar. This hot-stamped part has 22 holes and a total cutting length of 248 inches.
When this part went into production in 2005, a high-end, 5-axis laser system equipped with a 3.2-kW CO2 laser source cut it in 120 seconds. In 2007 a 5-kW CO2 laser source reduced cutting time to 78 seconds. The higher laser power made the plasma ignite and drastically increased cutting speeds to what is now known as high-speed cutting.
By 2009 the same part was cut in only 52 seconds, thanks in part to faster piercing, a process in which the machine ignites the laser when the cutting head is approaching the part. This piercing-on-the-fly reduced piercing time by up to 40 percent, especially on applications with multiple holes (see Figure 1).
Shortening Cycle Time
As demand for hot-stamped components has increased, so has the demand for any system that can perform postforming trimming operations. Today some 3-D laser cutting systems are designed specifically for processing hot-stamped parts.
To reduce part handling times, many systems have large rotary tables, a common configuration in hot-stamping applications. Another option is a dual-station configuration that has two tables placed side by side. A divider wall between the two splits the working area into two separate spaces. While the laser cuts the part, loading and unloading occur on the other side of the divider. This arrangement also makes it possible to set up a tool while a part is still in production. And if needed, the divider wall can be removed, opening up the entire working area for cutting larger parts.
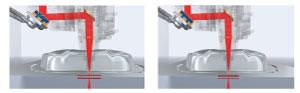
Figure 3: Today multiaxis cutting lasers can adjust the focal distance during the actual cutting process. These in-process adjustments allow for the efficient cutting of tailor welded or rolled blanks.
Consider one configuration with an open light curtain that allows the operator to access the rotary table from three sides. This improves part flow and reduces the walking distance between material bins and the table. A fully loaded, 13-ft.-diameter table can rotate in 2.3 seconds. A faster rotary table also stops faster, so the required safety distance from the light curtain to the table also can be reduced. This smaller light curtain area further reduces the footprint and decreases the walking distance for the operator. Moreover, the part’s high edge quality eliminates the need for deburring operations downstream.
All this shows how cycle times entail more than just a measurement of laser cutting speed. Time for cutting; part loading, handling inside the system, and unloading; as well as laser head positioning all help optimize overall cycle time.
The Solid-state Laser
Diode-pumped solid-state lasers have changed the 3-D cutting landscape. Such lasers emit a 1-micron-wavelength beam, and thin-sheet, hot-formed parts can absorb this wavelength more efficiently than the 10-micron wavelength produced by a CO2 laser. With higher beam absorption, a solid-state laser can achieve a faster cutting speed and higher- quality cut in thin sheets up to about 0.15 in.
The better incoupling of the wavelength also means a lower-power solid-state laser can be used. A 3-kW solid-state laser will cut faster than a 5-kW CO2 laser in thicknesses up to 0.075 in. Solid-state systems have higher wall-plug efficiency; more energy goes into the actual cut, and less is wasted. The systems require less maintenance and no laser gas. As for the assist gas, many have reduced costs further by using compressed air instead of nitrogen.
More Isn’t Always Better
For cutting 3-D hot-stamped parts, a higher laser power does not always result in faster processing. Consider a simple geometry versus a complex one requiring numerous contours and holes. If the material type and thickness are identical, the laser will cut the simple part faster. That’s because the complex part requires the laser head to reorient frequently, preventing the machine from reaching its maximum speed.
When processing parts that allow the system to cut at full speed over longer cuts, the higher laser power will yield shorter cycle times. On a complex part, a more powerful laser might reduce the cycle time slightly, but any gains usually are offset by the cost of the higher laser power (see Figure 2).
Typically, 3- and 4-kW solid-state lasers are used in cutting hot-stamped material. However, with a complex part less than 0.048 in. thick, a 2-kW laser has been shown to provide the best performance for the cost of operation.
Consistency and Quality
After processing a part, a manufacturer needs to confirm that all cuts have been completed successfully and that the slugs have been removed from holes. Conventional methods include visual part inspection, checking fixtures, or the capacitive distance control of the laser system’s cutting head. Certain solid-state 3-D laser systems, however, also have an integrated optical sensor for in-process measurement.
These systems can, for instance, measure the heat signature of a back reflection caused by a slug stuck in a hole. The cutting head moves over the laser-cut hole and fires the beam. If a slug is present, the machine reads the back reflection and provides the operator with a warning. Slugs tilted up to 45 degrees can be detected this way—a process that takes only 150 milliseconds, much faster than what’s possible with capacitive-distance sensing.
Another challenge in hot-formed applications is the ongoing trend to use tailor welded or rolled blanks with different thicknesses. Each area of the part has the exact material thickness and specifications required by the loads it is intended to withstand. This helps keep the overall part weight as low as possible.
For any laser-cut material, the focal point is set based on the material thickness. A clean cut can be achieved only with the correct focal-point distance in relation to the part surface. When faced with variable thicknesses within a part, manufacturers often choose the average value—a typical solution that actually makes the focal distance a compromise between the different thicknesses. The result can be poor cut quality overall.
Now, however, the focal distance can change during the actual cutting, so the correct parameters for all of the different thicknesses can be used. The point at which the focal distance changes is defined when the NC path is programmed. This helps produce a clean cut throughout the entire part. And because the adjustment is done in-process, the laser never slows down (see Figure 3).
Looking Forward
Laser cutting remains the most economical and viable production method for hot-stamped components. And to reduce scrap and processing time, manufacturers continually adapt and optimize their parts. For example, areas of a part with low tolerance requirements now are precut in the blanks, reducing the amount of laser cutting required.
However, processing systems have become too fast for a single operator, and reduced cycle times have created an issue for manual loading and unloading. Automation is the next logical step—and the future of multiaxis laser processing.
About the Author
Frank Geyer
Laser Technology Center 47711 Clipper St.
Plymouth Township , MI 48170
734-454-720
Related Companies
subscribe now
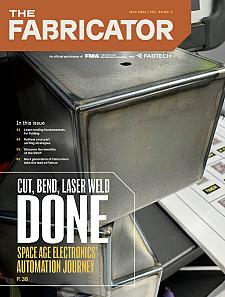
The Fabricator is North America's leading magazine for the metal forming and fabricating industry. The magazine delivers the news, technical articles, and case histories that enable fabricators to do their jobs more efficiently. The Fabricator has served the industry since 1970.
start your free subscription- Stay connected from anywhere
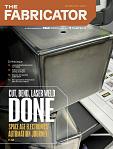
Easily access valuable industry resources now with full access to the digital edition of The Fabricator.
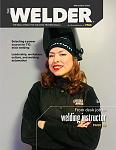
Easily access valuable industry resources now with full access to the digital edition of The Welder.
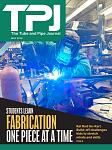
Easily access valuable industry resources now with full access to the digital edition of The Tube and Pipe Journal.
- Podcasting
- Podcast:
- The Fabricator Podcast
- Published:
- 04/16/2024
- Running Time:
- 63:29
In this episode of The Fabricator Podcast, Caleb Chamberlain, co-founder and CEO of OSH Cut, discusses his company’s...
- Trending Articles
Capturing, recording equipment inspection data for FMEA
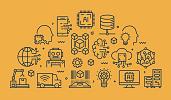
Tips for creating sheet metal tubes with perforations
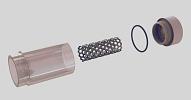
Are two heads better than one in fiber laser cutting?
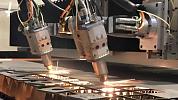
Supporting the metal fabricating industry through FMA
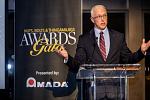
Omco Solar opens second Alabama manufacturing facility
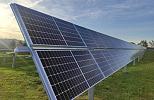
- Industry Events
16th Annual Safety Conference
- April 30 - May 1, 2024
- Elgin,
Pipe and Tube Conference
- May 21 - 22, 2024
- Omaha, NE
World-Class Roll Forming Workshop
- June 5 - 6, 2024
- Louisville, KY
Advanced Laser Application Workshop
- June 25 - 27, 2024
- Novi, MI