Business Development Manager
- FMA
- The Fabricator
- FABTECH
- Canadian Metalworking
Categories
- Additive Manufacturing
- Aluminum Welding
- Arc Welding
- Assembly and Joining
- Automation and Robotics
- Bending and Forming
- Consumables
- Cutting and Weld Prep
- Electric Vehicles
- En Español
- Finishing
- Hydroforming
- Laser Cutting
- Laser Welding
- Machining
- Manufacturing Software
- Materials Handling
- Metals/Materials
- Oxyfuel Cutting
- Plasma Cutting
- Power Tools
- Punching and Other Holemaking
- Roll Forming
- Safety
- Sawing
- Shearing
- Shop Management
- Testing and Measuring
- Tube and Pipe Fabrication
- Tube and Pipe Production
- Waterjet Cutting
Industry Directory
Webcasts
Podcasts
FAB 40
Advertise
Subscribe
Account Login
Search
New coil-applied nonmetallic coating protects press-hardenable steel
Unlocking process’s success at intersection of lightweighting and crashworthiness
- By William E. Fristad
- June 23, 2015
- Article
- Metals/Materials
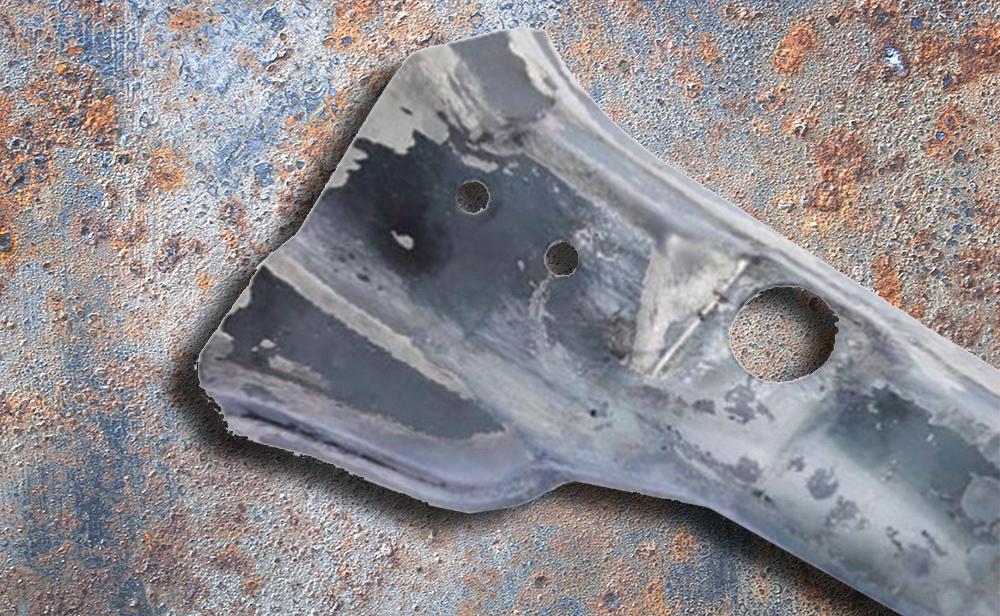
Scaling occurs on uncoated steel after it is heated to 900 degrees C. Coatings have been developed to eliminate or suppress scale.
Editor’s Note: This article has been adapted from a conference paper presented at the Steel Market Development Institute’s (SMDI) Great Designs in Steel 2014 conference in Livonia, Mich.
Press-hardening high-strength steel is an important component in automotive construction to provide crashworthiness (see Press Hardening Primer sidebar). It will become an even more critical component in body-in-white (BIW) construction as lightweighting becomes ever more important in meeting corporate average fuel efficiency (CAFE) guidelines for automotive OEMs.
If the steel is uncoated during the hot-stamping process, the final stamped part ends up with a thick layer of oxide scale caused by the reaction of the hot metal surface with atmospheric oxygen (see lead image). This scale must be removed by shotblasting, which can be costly and labor-intensive. Therefore, coatings have been developed to eliminate or suppress scale.
Several coating options are available today for the downstream use of stamped, press-hardened steel parts. However, none of these options are without limitations or challenges, such as cracking, coating transfer, fumes, weldability problems, and the need for shotblasting.
One approach is to use steel coated with an aluminum-silicon (AlSi) layer that is sacrificially oxidized and prevents significant oxidation of the underlying steel surface. This aluminized 22MnB5 steel is the current state-of-the-art substrate for hot stamping. Its advantages are that it suppresses surface scaling; does not require shotblasting; and is compatible with subsequent BIW cleaning, pretreatment, and e-coating processes. However, its disadvantage is that it is incompatible with indirect hot stamping because the aluminized layer cracks when cold. It also requires slow heating in the furnace so that iron in the steel has sufficient time to diffuse into the AlSi layer and raise the melting point of the new AlSiFe-alloy coating to prevent it from melting and transferring to contact points in the oven.
Another alternative is the use of a zinc-based coating—either zinc alone or zinc alloyed with nickel. This approach’s advantages are the cathodic protection the galvanized layer offers and that it is compatible with both direct and indirect hot stamping. However, zinc’s volatility and its migration into the steel, causing liquid metal embrittlement, are of significant concern.
A new approach to protecting press-hardenable steel during the hot-stamping process was sought.
Protective Coating Requirements
Efforts to develop a new protective coating centered on its meeting all key performance targets:
- Permanent
- Weldable
- Does not require shotblasting
- Hot-stampable without loss of adhesion
- Compatible with BIW pretreatment and e-coat
- Corrosion performance meeting
OEM paint specifications
Economics set the requirements for these key performance targets. If the hot-stamped part with a new coating still needed shotblasting, the coating would offer no value to what has already been developed. If it did not need shot- blasting but additional paints and coatings could not adhere to it, its value would be nil.
Having survived hot stamping, the coating must then be compatible with all normal downstream processing, including welding, cleaning, pretreatment, and painting. If the coated hot-stamped part is to be built into an autobody, it must perform during all the subsequent processes as well as traditional substrates.
To survive the high temperatures it is subjected to, the coating has to be primarily inorganic. To protect the steel from oxidization, it has to contain a sacrificially oxidized material.
New Coating Develops
A new coating technique has been developed and shows promise. The coating is a very thin (2- to 3-μm-thick), paint-like, rather than metallic, coating. It consists of a siloxane resin acting as the binder with aluminum flake to act as the sacrificial filler, so it meets the requirements to be inorganic and to contain a sacrificial oxidized material.
The coating is applied and dried on a standard coil coating line, similar to how paints are applied. A pretreatment is first applied to improve the coating’s adhesion and corrosion performance. The coated coil is then ready for blanking and hot stamping.
Trial Results
After undergoing several years of laboratory experiments, the coating formula was selected for scale-up and trials. The basic chemistries of the pretreatment and coil-applied coating have been manufactured on a full scale and applied to steel coils coated at a commercial coil coating line. Several steel producers and processors and hot-stamping firms are testing the product currently.
Characterization. At low magnification, the general smoothness of the coil-applied coating is visible, while at higher magnification, the presence of the aluminum flake within the coating starts to become evident (see Figure 1).
The coating can be analyzed by glow discharge optical emission spectroscopy (GDOES) to give an elemental depth profile through the coating (see Figure 2). The total oxygen content increased after heating because of the aluminum’s sacrificial oxidation and the iron increased within the coating layer. Thus, some iron has migrated into the 2-μm- thick coating.
Thermogravimetric analysis (TGA) shows the liquid coating as it would be applied to the steel coil (see Figure 3). The green curve shows the weight loss during a heating program from room temperature up to 1,000 degrees C. The largest weight loss occurs from room temperature to about 200 degrees C and is caused by solvent loss from the liquid coating. This weight loss would be seen during the curing of the paint on the coil line.
Once the coating is dried, the total weight loss from 300 to 1,000 degrees C is minor (3.3 percent) and occurs primarily in the 650- to 750-degrees-C range. It is within this range that the siloxane resin decomposes to more of a silica-like matrix.
Hot Stamping. The most important feature of the coating is that it must be capable of withstanding hot stamping. Blanks of the coated coils have been successfully hot-stamped (see Figure 4).
During the process of heating the press-hardenable, coated blanks, it was observed that the coil-applied-coated metal reached the targeted peak metal temperature considerably faster than AlSi-coated blanks used as a control. Under these “pizza oven” conditions, the heating time was reduced from seven minutes to three minutes. Because no melting or realloying occurred with the new coating, the temperature ramp-up to the peak metal temperature could be as rapid as possible, unlike the AlSi coating that requires controlled realloying with substrate iron. Thus, a shorter time in the oven was required for the component with the new coating than with the AlSi coating. This represents a significant energy cost savings.
It was also noted that the rate at which the heated blank started to cool down during the transfer from oven to die was slower for the coil-applied-coated blank than for the AlSi-coated blank, representing additional energy savings because the preheat temperature does not need to be as high.
Corrosion. First, the coated coils had to be stable during storage before being blanked and stamped or before being assembled into substructures or the BIW. A typical measure of storage stability is an accelerated humidity test (ASTM D1735-08). The coil-applied-coated steel showed no red rust developing after being exposed to 100 degrees F at 100 percent humidity for 168 hours. Hence, it is expected that coated coils or blanks will not rust while being stored before being hot-stamped.
Second, the hot-stamped parts must also be stable during storage after being hot-stamped and before assembly. When the parts, either coated with the new coating under testing or AlSi, were exposed to neutral salt spray (ASTM B-117) for 24 hours or 168 hours of humidity (ASTM D1735-08), spots of red rust corrosion were visible. These are consistent with small spots or defects in the coating where bare steel is exposed.
Analysis of causes for the rusting speculated that the coating may have thinned or disintegrated during the hot-stamping process, thereby exposing bare steel. Alternatively, iron could have migrated through the surface coating, allowing the iron to rust. Both coatings show comparable levels of corrosion (see Figure 5). The red iron oxide rust in the aluminized sample is caused by iron migration into the aluminized layer. The coating thickened to approximately 40 μm. The red rust visible in the 2-μm-thick coil-applied-coated samples could also be caused by iron migration or defects created during hot stamping.
Cleaning and Pretreatment. Hot-stamped parts must be compatible with downstream steps used in the BIW processing. These steps involve sequential alkaline cleaning, several water rinse stages, a pretreatment stage, additional rinsing, and electrocoating (e-coating). Today three different types of pretreatment are used commercially. Traditional zinc phosphating still is the most commonly used pretreatment. However, two newer pretreatments also have gained popularity. A process that applies a zinc phosphate coating to the steel and galvanized surfaces and a zirconium oxide pretreatment on aluminum components recently has been introduced. This pretreatment is done in a modified zinc phosphating bath followed by a second stage that deposits the zirconium oxide pretreatment. The third pretreatment being used commercially produces a zirconium oxide coating on all substrates. It goes by various trade names, but is often referred to as a thin film or nano-ceramic technology.
The first reaction to characterize was how the new coating was affected by typical alkaline cleaning. The as-coated (unheated) nonorganic liquid coating is hydrophobic because of the siloxane resin binder, and so this material behaved as expected. The coated surface emerged from alkaline cleaning and rinsing with a 100 percent water break surface, meaning that the water beaded up into droplets on the surface. This behavior is caused by the clean but hydrophobic surface, whereas, usually, the water break test is an indication of an oily metal surface that is not yet clean. Once the coil-applied-coated surface had been exposed to the high temperatures of hot stamping, the surface became very hydrophilic, or wet.
Thus, on the processing line, it is likely to act and look like all the other clean metal in the BIW.
The second step of the test was to examine the surface after the three different types of pretreatment. Because the hot-stamped surface was now coated with an AlSi oxide coating, essentially no metallic surface exists to react with the pretreatment solution. All three types of pretreatments rely on the acidic pretreatment solution reacting with a metallic surface, raising the pH in the immediate vicinity of the surface, and precipitating zinc phosphate or zirconium oxide. In the case of the coil-applied-coated surface, because there is no metallic surface, no reaction is possible, and therefore no deposition of zinc phosphate or zirconium oxide took place (see Figure 6).
Electrocoating. The third step of the BIW processing is deposition of a cathodic epoxy e-coat. It is necessary that a normal, defect-free, 20-μm-thick layer of e-coat be deposited on all the metal surfaces that might make up the BIW. When the hot-stamped part with the coating under testing was subjected to a standard e-coat bath and conditions, the same 20-μm-thick layer of paint was applied to both the press-hardened part as well as a hot-dipped galvanized part used as a control. E-coating of the coil-applied-coated part showed no other abnormalities such as pinholes or telegraphing. It had good adhesion.
Painted Corrosion Performance. The fully pretreated and e-coated part must meet standard corrosion requirements of the OEM. The corrosion trials showed that hot-stamped, coil-applied coated parts that have been e-coated had comparable performance to other e-coated steel parts subjected to salt spray, humidity, cyclic corrosion tests, and stone chipping.
Welding. The coated steel must be capable of being resistance spot-welded both before and after the hot-stamping process. In some cases, a blank has additional support pieces welded onto it before it is hot-stamped; and certainly after it is hot-stamped, the part needs to be weldable. The new coating presents a different surface with respect to electrical resistance. Before it is heated, the coil-applied coating is not particularly resistive; however, after being hot-stamped, it converts to a more resistive mixed-metal-oxide coating. Thus, the welding conditions before and after heating are expected to be different.
The coil-applied-coated steel samples, with and without the heat treatment, are being tested for resistance spot-welded conditions by an external laboratory. With the welding conditions developed (initially sloped current input, multiple pulses), near-expulsion-free welds were made. They exceed the AWS D8.1M and SEP 1220-2 minimum weld size, shear tension peak load of 18.2 kN, and cross tension peak load of 4.6 kN (see Figure 7). The new coating was not removed nor significantly damaged at the electrode-to-sheet interface during welding. Thus, its corrosion protection around the weld can be expected to be better than if it had been destroyed; however, this has not yet been verified.
Checklist
The current status of coil-applied coating is summarized as follows:
Successfully Completed:
- Full-scale manufacture of the liquid coating
- Full-scale application of the liquid coating on a coil coating line
- arts hot-stamped by several parties
- Parts e-coated after all three standard BIW pretreatment processes
Observed:
- Visually parts were equivalent to an aluminized steel control stamped at the same time.
- Heating time to peak metal temperature was less than with an aluminized steel control.
- Cooling rate upon exiting the oven before hot stamping was slower than with aluminized steel.
- Hot-stamped parts did not react with any of the three current pretreatment process types.
- Two coil-applied-coated parts could be resistance spot-welded to each other as well as welded to bare steel or hot-dipped galvanized.
In Development, Continuing:
- Coil-coated steel is tested by several steel companies
- Complete set of welding testing
- Testing to different OEM specifications and expectations
- BIW process studies to optimize corrosion performance
- Fine-tuning of the formulation to follow testing
Not only does the coil-applied coating prevent oxidation and scaling of the steel after it is heated in a furnace and hot-stamped, it appears to be compatible with downstream BIW construction, cleaning, phosphating, zirconium oxide pretreatment, and e-coating, and it is weldable, while providing corrosion protection. Although development of the entire process and product is not complete, initial trial results indicate that the coating shows promise of meeting all key requirements.
Press-Hardening Primer
A suitable way to accomplish both vehicle lightweighting and crashworthiness is to press-harden, or hot stamp, special steel alloys used to form the parts of an automobile body. The steel grade used is a manganese (1.0 to 2.5 percent) and boron (0.0005 to 0.004 percent) alloy often referred to as 22MnB5. Press-hardening steel requires the careful control of the steel crystalline structure by proper temperature control. The steel has excellent formability when heated above the austenitizing temperature—higher than 550 degrees C and typically about 900 degrees C.
When a hot-stamped part is quickly cooled at 50 to 100 degrees C per second in the die, the steel microstructure becomes martensitic and then has very high tensile strength, higher than 1,500 MPa. The very high strength is obtained only in the stamped part and not at the blanked stage. Thus, the unstamped steel can be easily processed at the blank stage.
The hot stamping may be done in either direct or indirect processes. In the direct process, the steel blank is heated in a stacked oven or roller hearth furnace to about 900 degrees C, transferred quickly to the die, and stamped while hot. In the indirect process, the blank is first stamped while cold to obtain either the final shape or the near-final shape, then heated and final-formed.
About the Author
William E. Fristad
32100 Stephenson Highway
Madison Heights, MI 48071
248-588-4719
subscribe now
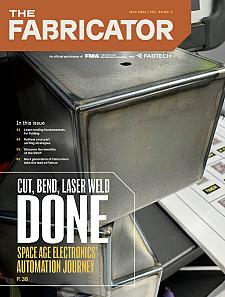
The Fabricator is North America's leading magazine for the metal forming and fabricating industry. The magazine delivers the news, technical articles, and case histories that enable fabricators to do their jobs more efficiently. The Fabricator has served the industry since 1970.
start your free subscription- Stay connected from anywhere
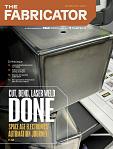
Easily access valuable industry resources now with full access to the digital edition of The Fabricator.
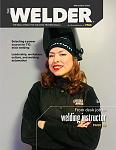
Easily access valuable industry resources now with full access to the digital edition of The Welder.
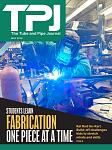
Easily access valuable industry resources now with full access to the digital edition of The Tube and Pipe Journal.
- Podcasting
- Podcast:
- The Fabricator Podcast
- Published:
- 04/16/2024
- Running Time:
- 63:29
In this episode of The Fabricator Podcast, Caleb Chamberlain, co-founder and CEO of OSH Cut, discusses his company’s...
- Industry Events
16th Annual Safety Conference
- April 30 - May 1, 2024
- Elgin,
Pipe and Tube Conference
- May 21 - 22, 2024
- Omaha, NE
World-Class Roll Forming Workshop
- June 5 - 6, 2024
- Louisville, KY
Advanced Laser Application Workshop
- June 25 - 27, 2024
- Novi, MI