Contributing Writer
- FMA
- The Fabricator
- FABTECH
- Canadian Metalworking
Categories
- Additive Manufacturing
- Aluminum Welding
- Arc Welding
- Assembly and Joining
- Automation and Robotics
- Bending and Forming
- Consumables
- Cutting and Weld Prep
- Electric Vehicles
- En Español
- Finishing
- Hydroforming
- Laser Cutting
- Laser Welding
- Machining
- Manufacturing Software
- Materials Handling
- Metals/Materials
- Oxyfuel Cutting
- Plasma Cutting
- Power Tools
- Punching and Other Holemaking
- Roll Forming
- Safety
- Sawing
- Shearing
- Shop Management
- Testing and Measuring
- Tube and Pipe Fabrication
- Tube and Pipe Production
- Waterjet Cutting
Industry Directory
Webcasts
Podcasts
FAB 40
Advertise
Subscribe
Account Login
Search
Metallurgy Matters: Reactions speak louder than words
- By Bob Capudean
- April 6, 2015
- Article
- Metals/Materials
Before we get into nonferrous gas-metal reactions, let’s look at one of the ways you can identify potential cold-cracking problems in steel. In the May/June 2004 issue, we looked at cold cracking—also known as hydrogen cracking, delayed cracking, and under-bead cracking—as it relates to hydrogen dissolved in steel. This problem is more prevalent in hardenable steels, which can transform to martensite in the heat-affected zone (HAZ) and weld metal.
That martensite can be a problem. Remember, martensite is extremely hard, even brittle. That hardness makes it susceptible to cold cracking if the metal can’t react to welding stresses. Add some carbon, which is responsible for the base metal’s hardness in the first place, and cold cracking becomes a real possibility. In fact, when dealing with carbon and low-alloy steels, generally resistance to cold cracking decreases as carbon and/or martensite content increases.
Carbon content is predetermined based on the formula for the steel you use. But what about martensite? What determines how much is formed in the weld metal and HAZ? Generally, that’s determined by the time spent at elevated temperatures and the cooling rate (the welding thermal cycle) along with the steel’s composition, especially carbon content.
Carbon has the most significant impact on cold cracking and weldability. But the other alloys used in the steel can also influence weldability and cold cracking. You can estimate the influence of other alloying elements by equating them to an equivalent amount of carbon. To do that, you use a formula to identify a carbon equivalent, or CE. The CE identifies the influence of total alloy content on the potential for cold cracking.
Finally, a Reason for Learning Algebra
Actually, it’s just arithmetic, but it looks worse than it is. The following are two different formulas for identifying a CE. Both formulas work. Which you use is based on whether your steel has phosphorus or vanadium in it. If it has both, use either:
You need to know how much of each specific alloy is in the steel. Generally, steels with low CE values have excellent weldability and aren’t prone to cold cracking. As the CE value increases, so doe the potential for problems.
CE values in the 0.20 to 0.30 range offer excellent weldability with virtually no susceptibility to cold cracking. But when values are higher than 0.40, hydrogen cracking becomes a real possibility. For example, most would consider steel with only 0.20 percent carbon (XX20) to have excellent weldability with no susceptibility to cold cracking. But 0.20 percent carbon steel with 1.60 percent manganese would have a CE of 0.60, suggesting a relatively high sensitivity to cracking (see Figure 1). Much to my chagrin, personal experience has proven this true.
Also keep in mind that cold cracking can happen only if all four of the following conditions are met:
- Atomic hydrogen must be present in the steel.
- The microstructure must be at least partly martensitic.
- A tensile stress must be present.
- The temperature must be less than 300 degrees F (150 degrees C).
Remember, if any one of these is not present, you’re safe—cold cracking can’t happen. By taking the appropriate preventive steps based on the CE for a given steel, you can go a long way toward ensuring at least one of those conditions is eliminated and your welds stay crack-free long after they’re done.
Gas-metal Reactions and Nonferrous Metals
As you might expect, our primary concern with nonferrous metal welds is the nemesis of steels welds—hydrogen—either as an element or in the form of water vapor.
Aluminum and Magnesium.A primary source of porosity, hydrogen usually gets into aluminum or magnesium weld pools thanks to hydrated oxides on the surface of the filler metal, workpiece, or both. Then as the metal solidifies, it rejects the hydrogen gas molecules. As that gas bubbles out and escapes, it leaves behind little holes known as porosity.
To prevent porosity, clean aluminum and magnesium filler metals and store them in airtight, sealed containers. Throwing in a couple of those little desiccant packs is also a good idea. Filler metals or workpieces exposed to moist air should be cleaned mechanically or with vacuum heating at 300 degrees F.
Copper and Copper Alloys. Similar problems can result when welding copper and copper alloys. Hydrogen combines with any oxygen in the weld pool to form water vapor, which causes porosity as the weld cools, although most copper filler metals contain deoxidizers to prevent this.
Zinc, beryllium, and aluminum alloys don’t have this problem because these elements are such stable oxides. Water vapor porosity can be a problem with nickel-copper and nickel-alloy weld metal, which is why filler metals designed for these alloys usually have strong deoxidizers.
Titanium. Hydrogen from the filler metal surface and soluble hydrogen in the filler or base metal are major causes of porosity in titanium welds, although practical experience has shown porosity to be the least of your worries when welding titanium.
The fact is, whenever you weld titanium, any area of the base metal that reaches 500 degrees F or higher must be protected from the atmosphere. To do that, you have two choices: either completely shield susceptible areas with inert gas, including the back side of the weld and the inside of tubing, or weld it in a vacuum.
The base metal must be protected because when titanium is heated to 500 degrees F or more, a number of gases, including nitrogen, hydrogen, and oxygen, react with it, causing embrittlement. And if that happens, you’ll know it by the amount of color you see in the finished weld. A god titanium weld is shiny silver to very light straw in color. Once you get more color than that, the weld is probably unacceptable. There are several visual inspection charts available that generally are a quick and effective way to judge the effectiveness of the shielding system.
That’s a quick look at gas-metal reactions in the welding of nonferrous metals. Next time we’ll talk briefly about solid-state reactions, which should be the perfect lead-in for a look at ways to strengthen weld metals.
About the Author
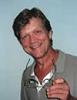
Bob Capudean
Back Alley Customs
About the Publication
subscribe now
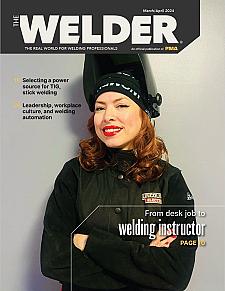
The Welder, formerly known as Practical Welding Today, is a showcase of the real people who make the products we use and work with every day. This magazine has served the welding community in North America well for more than 20 years.
start your free subscription- Stay connected from anywhere
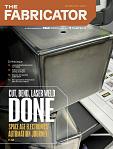
Easily access valuable industry resources now with full access to the digital edition of The Fabricator.
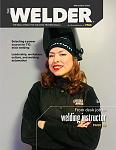
Easily access valuable industry resources now with full access to the digital edition of The Welder.
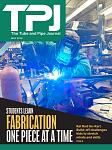
Easily access valuable industry resources now with full access to the digital edition of The Tube and Pipe Journal.
- Podcasting
- Podcast:
- The Fabricator Podcast
- Published:
- 04/16/2024
- Running Time:
- 63:29
In this episode of The Fabricator Podcast, Caleb Chamberlain, co-founder and CEO of OSH Cut, discusses his company’s...
- Trending Articles
Sheffield Forgemasters makes global leap in welding technology
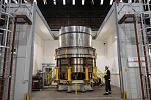
ESAB unveils Texas facility renovation
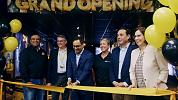
Engine-driven welding machines include integrated air compressors
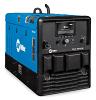
How welders can stay safe during grinding
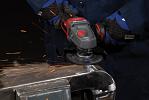
The impact of sine and square waves in aluminum AC welding, Part I
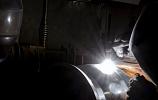
- Industry Events
16th Annual Safety Conference
- April 30 - May 1, 2024
- Elgin,
Pipe and Tube Conference
- May 21 - 22, 2024
- Omaha, NE
World-Class Roll Forming Workshop
- June 5 - 6, 2024
- Louisville, KY
Advanced Laser Application Workshop
- June 25 - 27, 2024
- Novi, MI