Regional Sales Managers
- FMA
- The Fabricator
- FABTECH
- Canadian Metalworking
Categories
- Additive Manufacturing
- Aluminum Welding
- Arc Welding
- Assembly and Joining
- Automation and Robotics
- Bending and Forming
- Consumables
- Cutting and Weld Prep
- Electric Vehicles
- En Español
- Finishing
- Hydroforming
- Laser Cutting
- Laser Welding
- Machining
- Manufacturing Software
- Materials Handling
- Metals/Materials
- Oxyfuel Cutting
- Plasma Cutting
- Power Tools
- Punching and Other Holemaking
- Roll Forming
- Safety
- Sawing
- Shearing
- Shop Management
- Testing and Measuring
- Tube and Pipe Fabrication
- Tube and Pipe Production
- Waterjet Cutting
Industry Directory
Webcasts
Podcasts
FAB 40
Advertise
Subscribe
Account Login
Search
Fiber laser technology making inroads into tube, pipe market
Why the low-maintenance tech is suited to make seam welds for tube and pipe production
- By Kevin Arnold, P.E.
- October 17, 2019
- Article
- Tube and Pipe Production
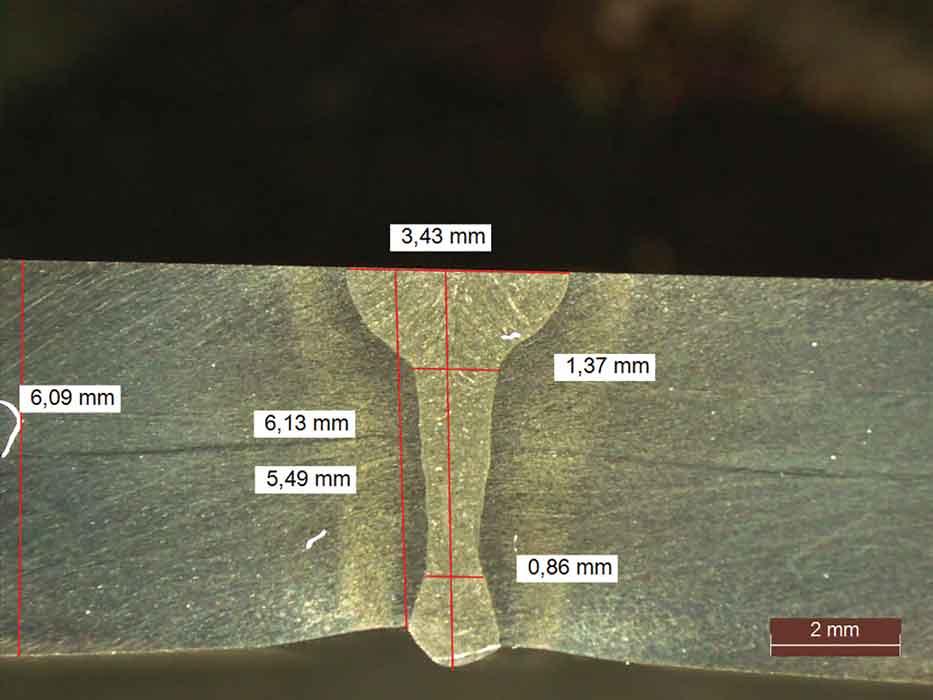
With very high beam quality and a variety of focusing optics, fiber laser technology provides a narrow, low-heat-input weld that results in a nail-like cross section, providing optimal strength, ductility, and formability. Fiber laser welding is well-suited to modern materials such as the very-high-strength steels that are growing in prominence in the automotive industry.
For every new technological innovation, another follows. Some technologies emerge and carve out a niche for themselves, dividing the market; others displace the preceding technology, rendering it obsolete, and take over the market.
Among electrical and electronics products, a new type of technology often supplants an old one. The incandescent light bulb is essentially a thing of the past, replaced by compact fluorescent, halogen, and light-emitting diode varieties. Vinyl records, cassette tapes, and compact discs have been nearly replaced by electronic files. Film cameras are favored among some diehards, but the market essentially has been taken over by digital cameras. A half century ago, radios and amplifiers based on vacuum tubes were pushed out by solid-state units, modern products based on semiconductors.
For many decades, the market for industrial lasers was dominated by the CO2 variety. When applied to a tube or pipe mill and used for welding the seam, the CO2 laser carved out a niche as a contender, a viable alternative to resistance and arc welding processes. These days, the CO2 variety is losing ground to another technology: solid-state lasers.
A Disruption in Welding
Depending on the application, tube and pipe producers have made use of gas tungsten arc welding, high-frequency electric resistance welding (HF-ERW), plasma arc welding, and laser welding. For a handful of niche applications, such as stainless steel umbilical lines used thousands of feet below sea level, laser welding provides superior results, creating welds with integrity that the others can’t match.
However, as with nearly every technology, laser welding comes with some drawbacks. First, it’s not as fast as the most common tube and pipe mill welding process, HF-ERW. Second, the laser light requires more stringent guarding than the arc welding processes to protect operators from reflected light. Third the small spot size means that keeping the weld seam at the 12:00 o’clock position is critical. Finally—not really a drawback, but a basically a draw—is that the investment doesn’t offer much in the way of a financial benefit. The capital cost of a laser welding unit is on par with that of an HF-ERW unit.
However, a host of benefits make this a technology worth investigating.
CO2 Versus Solid-state Lasers
As the name suggests, the incumbent CO2 laser uses a gas mixture based on carbon dioxide. The mix of CO2 and other gases, commonly nitrogen and helium, is electrically excited to create the welding beam. Some lasers have a turbine, tangential, or roots-style blower to move that gas past electrodes excited by either a radio frequency signal or direct current, while some have a static gas mixture that is exchanged after some specified number of hours of operation. All of the gases must be evacuated from the resonator and replenished according to a schedule, which leads to consumable, facility, and labor costs to store and maintain them. As helium becomes increasingly scarce, this commodity gas will cause ever-increasing operational costs, as well as management of its gas supply sources and others at the mill site.
CO2 lasers emit light energy in the far infrared spectrum at a wavelength of 10,600 nanometers (nm), which also is referred to as 10 μm. This light must be directed from the laser source to the workpiece by a series of encapsulating tubes for straight shots and mirrors that change its direction. Proper maintenance requires scrupulous attention to a cleaning schedule and regimen to prevent damaging the highly reflective, polished copper surfaces of the mirrors, which normally are treated with special coatings. Another requirement is that they must be kept in alignment both axially and orthogonally; that is, kept at right angles at each bend location to keep the beam centered in the delivery tube so that the focused beam hits the desired location on the tube’s seam. This complex beam delivery requirement is subject to vibrations on the shop floor and seasonal changes in temperature and humidity.
Solid-state lasers come in fiber or disk varieties and are available in the same power ranges as their CO2 counterparts. Like the CO2 variety, the name is descriptive, identifying the laser beam’s origin as a solid, either an extruded glass fiber or a small crystalline disk. Both have a rare-earth element suspended, or doped to use the industry term, in the solid matrix that is excited by diodes to emit light in in wavelengths from 900 to 1,070 nm (commonly called 1 µm).
A key advantage of this wavelength, which is one-tenth of the CO2 wavelength, is that it can be transported from the laser resonator to the welding head via a flexible fiberoptic cable. Therein lies one of this technology’s biggest advantages: a very simple beam delivery system that doesn’t have a cleaning schedule or an alignment procedure. This eliminates a thankless task and regular downtime—a win for the people on the shop floor and for the operations manager. This alone serves as a “Eureka!” moment on the shop floor and in the office area. For many tube and pipe producers, drastically cutting the necessary maintenance time boosts productivity to the point that it justifies the upgrade. For their part, fiber lasers have no user-serviceable parts and do not have a monthly or even an annual maintenance requirement, other than simply changing the water for the chiller that cools the laser and focusing optics.
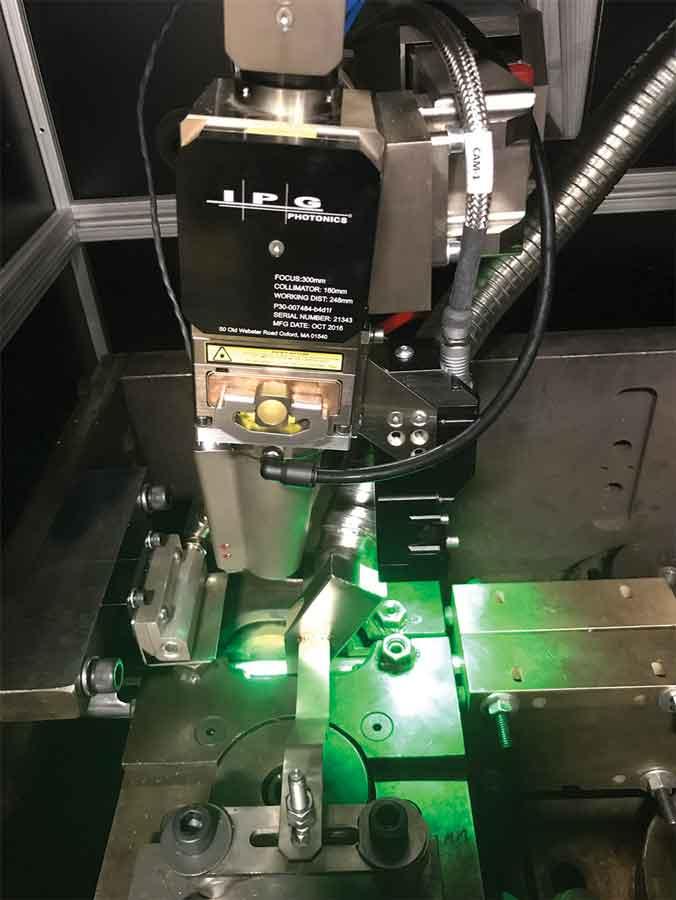
Superior Roll Forming Co., Valley City, Ohio, upgraded a strategic seating component line with an 8-kW IPG fiber laser to improve production capability and weld quality. Photo courtesy of Superior Roll Forming Co.
Solid-state Lasers on Solid Footing
From a facilities standpoint, solid-state lasers do not require nearly as many resources as their CO2 counterparts. Beyond the simplified beam delivery system, possibly of greater value is the modest floor space requirement. These days fiber lasers from 8 to 10 kilowatts (kW) require just 20 percent of the floor space of CO2 lasers of similar power. Add the fiber laser’s electrical efficiency, which is 3 to 5 times better than that of CO2 lasers, and the hourly operating costs are much less than those of CO2 technology. Finally, solid-state lasers require no gases to generate the beam. The chief concern here is helium, the supply of which is finite. The cost is rising, and in some cases the supply is rationed.
With any laser technology, operator safety is a primary concern. Light reflected from the laser source damages retinas quickly because they readily absorb laser wavelengths. As mentioned already, the CO2 beam must be transmitted from the source to the weld box in tubes to prevent the invisible light inside from coming in contact with personnel. The fiber cable of a solid-state laser is protected in multiple layers for durability and safety and is an ideal light-transport device. Eye protection for CO2 lasers can be almost any glass or plastic material, since either will absorb much of the reflected light and prevent it from reaching the eye. Solid-state lasers require eye protection coated with wavelength-specific materials that absorb any light reflected from the process. Both laser types require an ANSI Class 1 enclosure to ensure that any reflected light that escapes from the weld box is below the acceptable threshold.
Capabilities and Caveats. In general laser welding is used on stainless steel tubing, but it’s suitable for nearly any ferrous or nonferrous alloy. When optimized, solid-state lasers can weld tube with a very thin wall and very small diameter, such as hypodermic product, up to a thick wall of about 5/16 inch (8 millimeters).
While laser power and line speed correlate, this doesn’t mean that increasing the line speed is just a matter of increasing the laser’s power. On one hand, the practical limit is usually from 33 to 49 feet per minute (10 and 15 meters per minute) to prevent irregularities in the top bead (also known as humping). On the other hand, if the top bead will be ground, planished, scarfed, or otherwise cosmetically treated, then these wave-like solidification patterns may be ignored, allowing the line to run faster.
Laser welds usually have very parallel sides and lead to very good formability compared to forging and resistance welding techniques. This has been the primary advantage in welding 400 series stainless tubing for exhaust systems that require small-radius bends and forming into bellows shapes for thermal expansion and contraction control.
Solid-state lasers produce a beam that can be focused to a smaller spot diameter than many CO2 lasers. This can be a substantial advantage, but only when the tube mill can present the seam at top dead center with good edge preparation. If the spot size is too small, however, excessive weld pool volatility can cause missed seams and weld spatter in the OD or ID. The shorter wavelength helps most steels absorb the laser’s energy more efficiently than CO2 lasers, so care must be taken to get the desired quality from the weld process. CO2 lasers have a good reputation for clean welds inside and outside of the tube, but much work has been done in the past several years to allow solid-state lasers to produce similar results. Today the results are essentially identical.
Laser welding with fiber lasers frequently benefits from welding with the strip edges’ burr facing down. Controlling the vertical and horizontal gap at the pinch point to 5 percent or less of the strip thickness also pays dividends of high weld quality and appearance. Optimizing the focus position relative to the pinch point as well as focusing, usually on the strip surface, helps ensure consistent good quality and minimize scrap.
Recent advances in camera and digital signal processing have made optical seam tracking a robust subsystem that contributes to a high-quality welded seam. A camera detects the strip’s gap near the squeeze rolls to calculate the optimal position for the focusing head as the weld seam’s position wanders. Mills also can have additional cameras that show the top bead so operators can look for weld defects like undercut, underfill, and lack of fusion.
An Application Illustration. A typical application for solid-state laser welding, automotive components, illustrates the scope of this technology’s role now and in the foreseeable future.
As weight reduction in vehicles becomes increasingly important, welding of high-yield-strength materials for structural elements is becoming more common. In many cases, legacy laser welding systems are brought back online to weld these parts. Operators facing large work volumes are looking at the older CO2 laser systems with some suspicion, because many are ending their usable lifespans. Faced with possible unplanned downtime halting production to repair a very complex laser system or replacing with current technology, some tube and profile producers are turning to solid-state laser systems to help cut production costs, improve line uptime and reliability, and cut utility and consumable costs. This translates into lower cost per part and higher profitability.
A cost justification for a new line, or updating an existing line, should include the following considerations:
The current state of product support from the laser OEM
Availability of spare parts and trained service technicians to install them
History of the existing laser and any outstanding or ongoing maintenance issues
Process improvements relative to a solid-state laser
Floor space savings for the solid-state laser and chiller
Eliminating gas storage at the laser
Eliminating laser radio frequency power supply and other electrical cabinets
Eliminating vibration isolation for the laser and beam delivery
Reducing spare parts inventory
Fiber cable for the laser and filters for the chiller
Replacement lenses and optical elements for the welding focus head
Reducing power consumption and eliminating consumables
Consuming 10 percent of the electricity of a conventional laser
Eliminating the CO2, helium, nitrogen, and other gases to generate the beam
Eliminating gases or air drier for beam delivery tubes
Eliminating consumable optics in the beam path
A Path to the Future
Considering all the main and peripheral factors, the case for a solid-state laser is easy to make. The enabling nature of the 1-µm wavelength solid-state fiber laser enhances tube, pipe, and profile production in technology and efficiency. The benefits that result often contribute to a return on investment of one to three years.
Any material that can be fusion-welded can be laser-welded, so as the technology improves in power, reliability, and optics, the many companies involved in making tube, pipe, and profile will continue to benefit and work on the foundation laid by the CO2 pioneers.
Kevin Arnold, P.E., is Midwest sales manager for IPG Photonics, 50 Old Webster Road, Oxford, MA 01540, 877-980-1550, karnold@ipgphotonics.com, ipgphotonics.com.
About the Author
Kevin Arnold, P.E.
50 Old Webster Road
Oxford, MA 01540
877-980-1550
About the Publication
Related Companies
subscribe now
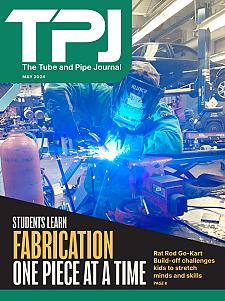
The Tube and Pipe Journal became the first magazine dedicated to serving the metal tube and pipe industry in 1990. Today, it remains the only North American publication devoted to this industry, and it has become the most trusted source of information for tube and pipe professionals.
start your free subscription- Stay connected from anywhere
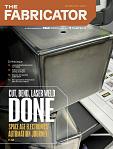
Easily access valuable industry resources now with full access to the digital edition of The Fabricator.
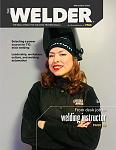
Easily access valuable industry resources now with full access to the digital edition of The Welder.
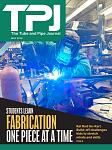
Easily access valuable industry resources now with full access to the digital edition of The Tube and Pipe Journal.
- Podcasting
- Podcast:
- The Fabricator Podcast
- Published:
- 04/16/2024
- Running Time:
- 63:29
In this episode of The Fabricator Podcast, Caleb Chamberlain, co-founder and CEO of OSH Cut, discusses his company’s...
- Trending Articles
Zekelman Industries to invest $120 million in Arkansas expansion
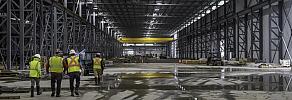
3D laser tube cutting system available in 3, 4, or 5 kW
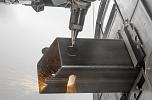
Corrosion-inhibiting coating can be peeled off after use
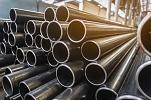
Brushless copper tubing cutter adjusts to ODs up to 2-1/8 in.
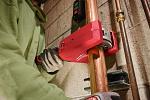
HGG Profiling Equipment names area sales manager
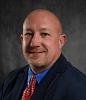
- Industry Events
16th Annual Safety Conference
- April 30 - May 1, 2024
- Elgin,
Pipe and Tube Conference
- May 21 - 22, 2024
- Omaha, NE
World-Class Roll Forming Workshop
- June 5 - 6, 2024
- Louisville, KY
Advanced Laser Application Workshop
- June 25 - 27, 2024
- Novi, MI