Contributing Writers
- FMA
- The Fabricator
- FABTECH
- Canadian Metalworking
Categories
- Additive Manufacturing
- Aluminum Welding
- Arc Welding
- Assembly and Joining
- Automation and Robotics
- Bending and Forming
- Consumables
- Cutting and Weld Prep
- Electric Vehicles
- En Español
- Finishing
- Hydroforming
- Laser Cutting
- Laser Welding
- Machining
- Manufacturing Software
- Materials Handling
- Metals/Materials
- Oxyfuel Cutting
- Plasma Cutting
- Power Tools
- Punching and Other Holemaking
- Roll Forming
- Safety
- Sawing
- Shearing
- Shop Management
- Testing and Measuring
- Tube and Pipe Fabrication
- Tube and Pipe Production
- Waterjet Cutting
Industry Directory
Webcasts
Podcasts
FAB 40
Advertise
Subscribe
Account Login
Search
MIAB welding of thick-walled pipe ends
Research finds ways to increase pipe thickness while maintaining quality
- By Dr. Vladimir Kachinskiy
- July 13, 2004
- Article
- Tube and Pipe Production
Various methods are used for welding pipe, including electric shielded metal arc welding (SMAW), submerged arc welding (SAW), and flash end welding.
Another efficient process is magnetically impelled arc butt (MIAB) welding. Current technology and equipment allow MIAB welding of pipes with wall thicknesses to 6 millimeters and diameters up to 219 mm. This process has been applied for thin-walled pipe used in the automobile, machine building, and construction industries, among others.
In recent years the E.O. Paton Electric Welding Institute, Ukraine, has investigated new applications for MIAB welding, including thick-walled pipe and solid workpieces. This article discusses the results of testing MIAB welding of pipes with wall thicknesses up to 16 mm.
Controlling Arc Movement in the Gap
In MIAB welding, an external, controlled magnetic field moves the arc in the gap between the pipe edges (see Figure 1). Two pipes ready for welding are set coaxially. Magnetic systems installed opposite each other form magnetic fluxes in the arc gap. This magnetic field in the arc gap consists of two vector components of magnetic induction, B: radial (Br) and axial (Ba).
A short circuit excites the welding arc. The pipes to be welded are moved apart for a definite arc gap (1.5 to 2.5 mm). The interaction (see Figure 2) between the welding arc's axial component of current and a radial magnetic component, directed perpendicular to the welding arc current, leads to the creation of force. This force moves the welding arc along the ends of the pipes.
![]() |
Figure 1 Two pipes are set coaxially in MIAB welding, and an external, controlled magnetic field moves the arc in the gap between the pipe edges. |
MIAB welding uses a preprogrammed control of arc current, with arc movement that can reach a linear speed of 200 meters per second. This control heats the pipe ends uniformly, thus producing a quality welded joint.
Conventional MIAB welding on pipes thicker than 6 mm cannot meet the required welded joint quality, mainly because the welding arc column active spots should be proportional to the pipe end thickness for uniform and stable heating.
The traces of the arc column active spots are smaller than the cross section of the pipe ends. The welding process is unstable, with short-circuiting. Under the action of the welding arc's natural magnetic field and a high gradient of the external magnetic field induction, the arc is forced out from the arc gap at the initial moment and moves on the inside edges of the pipe ends. The arc column is bent to the side of a geometric axis of the pipe.
As the inside end edges heat up, the gradient of distribution of the magnetic field induction in the arc gap decreases, and the welding arc shifts to the outside edges. An 8-mm-thick pipe wall prevents stable movement of the arc to the area of a higher controlled magnetic field induction value. The unstable movement of the welding arc in the area with a low induction value leads to a nonuniform heating of the pipe ends.
The result is an overheating area covering up to 70 percent of the heated section of the inside pipe edges. After upset, this area of overheating remains in a central part of the welded joint, thus negatively influencing the welded joint's properties.
![]() |
Figure 2 Several individual forces produce a resultant force that displaces the arc during MIAB welding: |
B = induction of the magnetic field Br = radial component Ba = axial component I = arc current Ia = axial component Ir = radial component FBa = force that affects the radial displacement of the arc FBr = force that affects the displacement of the arc |
Research into MIAB welding of thick-walled pipes has focused on control methods that will make it possible to move the arc over the entire area of the cross section of the pipe ends.
Initial research also targeted the movement of the welding arc to the outside edges of the pipe ends via the optimum distribution of the magnetic field induction in the arc gap. With this movement, the welding arc's heat energy is distributed at the edges uniformly, but at a lower welding current density over the larger area of the welded section than that occupied by the active spots of the arc column. This results in uniform heating of the pipe ends as compared with heating from movement of the welding arc on the inside pipe edges.
The welding arc, controlled by the magnetic field, is shifted to the outside edges of the thick-walled pipe ends, in the area of a maximum value of the magnetic field induction. The movement of the welding arc on the outside edges of the thick-walled pipes can produce uniform heating.
With the new MIAB welding process, the overheating area covers up to 30 percent of the heated section in the area of the outside edges. After upset, the overheated area is pressed out into a flash and reinforced.
In the new process, interaction of the radial component of the electric current with the axial component of the magnetic field induction induces force that causes displacement of the arc to the outside edges. Pipe ends are heated as the welding arc moves along the outside edges. When required heating is reached, the forces and the welding current value are changed, causing the welding arc to scan along the surface of the ends. Then the upset is performed.
Formation of Joints in Thick-walled Pipes
The uniform heating of pipe edges is basic, but not a sufficient condition for the formation of a quality joint. Since shielding gases usually are not used in MIAB welding, pipe ends heated with oxygen or air can oxidize, and these oxides must be removed.
Experiments established that it is feasible to achieve the arc rotation frequency required to make quality welds on pipes up to 16 mm thick. On these pipes, shielding is created in the gap space between the parts as a result of intensive metal evaporation, preventing the oxidation of melted metal areas. The joints are formed in the liquid phase without any defects.
Figure 3 shows the macrosection of the welded joint of a 76-mm-diameter, 16-mm-wall-thickness steel pipe. Metallographic examinations of this welded joint were carried out after etching in a 4 percent solution of HNO3 in alcohol, and measurements were made in different parts of the welded joint. In area 2 the structure of the weld line is ferritic; its width is 0.01 mm.
The structure of area of the complete recrystallization is a fine-dispersed ferritic-pearlitic mixture. Structure of the area of the partial recrystallization is the ferrite-pearlite.
The microstructure in area 3 of Figure 3 is in transition. The width of the weld line is 0.0252 mm; the structure is ferritic. The HAZ consists of an area of overheating, complete recrystallization, and partial recrystallization. The structure is ferritic-pearlitic.
During heating the overheating area is formed in the spots of the burning welding arc. During the upset, the coarse-grain area is pressed out into pre-external reinforcement and flash, and the welded joint is formed at the expense of the fine-grain area.
The new MIAB welding process for thick-walled pipes has no strict requirements for pipe end preparation. Welding time is short, from 10 to 100 seconds. Heating around the end perimeter is uniform and concentrated, with minimal allowances for flashing and upset.
The automated process requires no welding consumables or shielding gas, and it results in welds that are free from pores, inclusions, or volume defects.
Dr. Vladimir Kachinskiy is senior staff scientist at E.O. Paton Welding Institute, 11 Bozhenko St., Kyiv, Ukraine 03680, +380442615018, fax +380442274254, kachinskiy@paton.kiev.ua; Dr. Valeriy Krivenko is director of the Pressure Welding Engineering Centre, E.O. Paton Welding Institute, 26 Raketnaya St., Kyiv, Ukraine 03028, +380442647304, fax +380442646901, elweld@viaduk.net.
About the Author
About the Publication
subscribe now
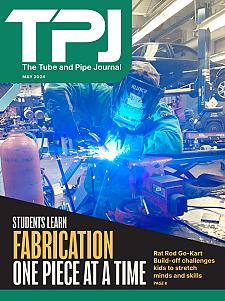
The Tube and Pipe Journal became the first magazine dedicated to serving the metal tube and pipe industry in 1990. Today, it remains the only North American publication devoted to this industry, and it has become the most trusted source of information for tube and pipe professionals.
start your free subscription- Stay connected from anywhere
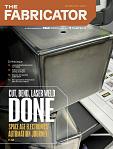
Easily access valuable industry resources now with full access to the digital edition of The Fabricator.
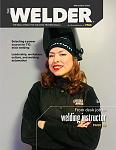
Easily access valuable industry resources now with full access to the digital edition of The Welder.
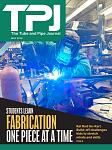
Easily access valuable industry resources now with full access to the digital edition of The Tube and Pipe Journal.
- Podcasting
- Podcast:
- The Fabricator Podcast
- Published:
- 04/16/2024
- Running Time:
- 63:29
In this episode of The Fabricator Podcast, Caleb Chamberlain, co-founder and CEO of OSH Cut, discusses his company’s...
- Trending Articles
Zekelman Industries to invest $120 million in Arkansas expansion
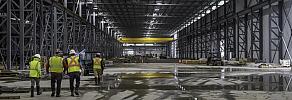
3D laser tube cutting system available in 3, 4, or 5 kW
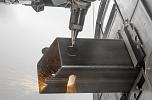
Corrosion-inhibiting coating can be peeled off after use
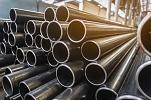
Brushless copper tubing cutter adjusts to ODs up to 2-1/8 in.
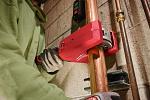
HGG Profiling Equipment names area sales manager
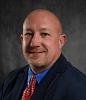
- Industry Events
16th Annual Safety Conference
- April 30 - May 1, 2024
- Elgin,
Pipe and Tube Conference
- May 21 - 22, 2024
- Omaha, NE
World-Class Roll Forming Workshop
- June 5 - 6, 2024
- Louisville, KY
Advanced Laser Application Workshop
- June 25 - 27, 2024
- Novi, MI