Founder
- FMA
- The Fabricator
- FABTECH
- Canadian Metalworking
Categories
- Additive Manufacturing
- Aluminum Welding
- Arc Welding
- Assembly and Joining
- Automation and Robotics
- Bending and Forming
- Consumables
- Cutting and Weld Prep
- Electric Vehicles
- En Español
- Finishing
- Hydroforming
- Laser Cutting
- Laser Welding
- Machining
- Manufacturing Software
- Materials Handling
- Metals/Materials
- Oxyfuel Cutting
- Plasma Cutting
- Power Tools
- Punching and Other Holemaking
- Roll Forming
- Safety
- Sawing
- Shearing
- Shop Management
- Testing and Measuring
- Tube and Pipe Fabrication
- Tube and Pipe Production
- Waterjet Cutting
Industry Directory
Webcasts
Podcasts
FAB 40
Advertise
Subscribe
Account Login
Search
Combatting corrosion in piping installations
Understanding the causes and preventing the occurrence of corrosion in all of its forms
- By William A. Byrd, P.E., C.S.
- May 26, 2017
- Article
- Metals/Materials
For all of the damage corrosion does and the difficulty manufacturers have in preventing it, the process by which corrosion develops isn’t a mystery. While it can take several forms, corrosion usually can be traced to a single cause: Energy flowing from one material to another.
Everything contains energy, and we know from the study of physics, specifically the laws of thermodynamics, that energy cannot be created or destroyed. It is ever-present, in the exact amounts that it has always been present in the universe. Einstein’s famous equation E=mc2 provides the relationship between matter and energy.
Energy just moves from place to place, and when it is stored, it is always trying to escape. The more energy we put into a material, such as steel through the foundry processes, the more pressure energy has on it to escape. For example, we heat ferrous oxide, or iron ore, to a very high temperature to create steel. This heat energy is stored inside the steel and is just waiting to get out. It can use numerous escape methods, including friction, or heat; chemical, when exposed to attacks from oxidizers; atmospheric, when rusting in open air; and electrical, when steel becomes the sacrificial anode in an electrical circuit.
This last one, electrical, is worth studying. All metals and other materials can be found on an available energy chart called the electromotive series (galvanic series), which provides the electrical energy relationships among metals (see Figure 1). Remember, metals are created from ore—basically dirt—and are always trying to escape and return to dirt. The amount of energy we put into making each metal, and the differences of these energy inputs among metals, determines the position of each on the electromotive series chart. The higher up the chart, the greater the stored energy; the further down, the less the stored energy. For example, iron and steel are higher on the chart than copper, which is understandable, because copper can be made from low heating of copper ores, while steel takes much more heat to create from iron ore. That’s why the Bronze Age, which was based on using a copper alloy, came before the Iron Age.
When these two metals, copper and iron, are connected together by wire or direct contact and then placed into an environment that completes an electrical circuit between them, such as earth, energy will escape from the high-energy metal (iron) to the lower-energy metal (copper) (see Figure 2). This creates an electrical voltage and is how batteries are made.
When a circuit is created, high-energy metals become electrically positive (anodes) relative to the low-energy metals, which become electrically negative (cathodes). The anode corrodes as its energy escapes to the cathode. The cathode receives energy and is protected against corrosion, or energy loss, by the corroding anode. In other words, the steel anode is sacrificing its energy to the copper cathode. This electrical energy difference is approximately 0.07 volt when measured between a copper cathode and new steel.
Using high-energy metals to protect low-energy metals is the basis for what is called cathodic protection. If we connect a high-energy metal such as zinc to a lower-energy metal such as steel, then complete the electrical circuit in soil or water, the zinc will corrode, protecting the steel from corrosion. This is protection of the cathode, steel, by sacrificing the anode, zinc.
This is why steel pipelines should have sacrificial anodes electrically attached to them when they are placed into the ground, completing electrical circuits. The anodes give up energy to protect the steel and the steel will not corrode, but the anode will corrode and will have to be replaced when its energy is depleted.
Corrosion Types
Several types of corrosion, or energy loss, typically are found with materials in various applications. Corrosion is categorized by the specifics that start and sustain the corrosion process.
Galvanic Corrosion. Galvanic corrosion is the most common corrosion. A typical example is a grounding clamp on a piping system in a residence or a commercial building. In this scenario, a copper wire is connected to a steel pipe to ground it. Humid air acts as an electrolyte. Because both the copper wire and the steel pipe are in contact with soil outside the building, this system is a complete circuit. Copper and steel have an energy differential of about 0.7 V.
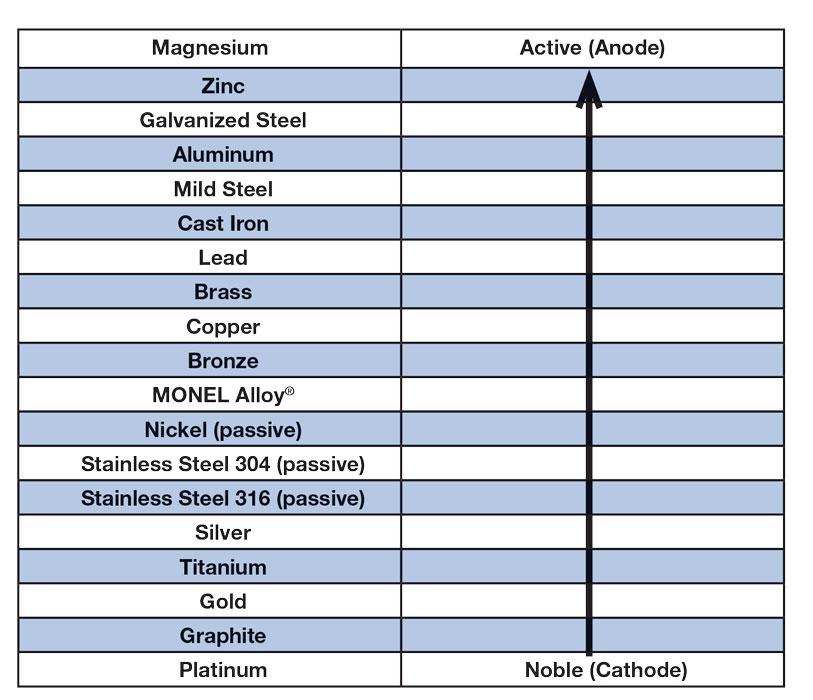
Figure 1
Typical electromotive (galvanic) series relationships between a few metals are shown here. Every element has an electromotive force, which gives every alloy an inherent voltage level. When two alloys make contact, current flows from the high-energy metal to the low-energy metal.
Galvanic corrosion can occur in many forms and in varying severity, which is a matter of the energy difference. An unusual example of this can occur when two pipelines are made from the same materials but have different temperatures. Under the right conditions, the relatively hot pipe acts as an anode, corroding and protecting the relatively colder pipe. Another unusual example occurs when two pipes, made from one material but different ages, are welded together. The newer of the two pipes has more energy and corrodes, thereby protecting the older pipe from corrosion.
Anodic Corrosion. Stray DC currents cause most instances of anodic corrosion. This can happen anytime a pipeline is exposed to DC energy flowing through the ground. A common scenario is a pipeline that runs near a DC-powered train or trolley.
Cathodic Corrosion. Cathodic corrosion or alkaline corrosion can occur at the cathode, depending on the materials involved. The most common type of alkaline corrosion damages alkaline batteries. Interestingly, alkaline water and some alkaline conditions actually protect steel pipe from corrosion by forming a protective film of ferric hydroxide.
Chemical Corrosion. Chemical corrosion is caused most often when an acid contacts a metal. This can happen in chemical plants, paper mills, and petroleum refining facilities. A common form of chemical attack occurs under the insulation of a pipeline.
AC Electrical Corrosion. AC electrical corrosion is caused by induction, usually when pipelines run parallel to high-voltage electrical lines (see Figure 3). The pipe intercepts the AC current, which then travels along the pipe. Eventually the current returns to its source through the soil, completing the circuit. Often the current leaves the pipe and enters the soil where the pipe changes direction; this is where the corrosion occurs.
Local Cell (Pitting) Corrosion. Local cell or pitting corrosion can occur on any metal. It is the main form of corrosion attack on aluminum and some types of stainless steels. These metals normally are protected by a hard, thin film that forms at the surface when they are exposed to oxygen (aluminum oxide and chromium oxide, respectively). Pitting corrosion develops when the protective film is penetrated or removed by an electrical, chemical, or mechanical process.
Crevice Corrosion. Crevice corrosion occurs in protected or shielded areas in pipelines that can capture or create a corrosive environment.
Stress Crack Corrosion. Stress cracking is actually a form of corrosion. It occurs in nearly all metals and most often in high-strength materials. The fracturing is normally along grain boundaries and can be intergranular in nature.
Steels that are stressed beyond grain boundary limits are very susceptible to corrosion attack in severe environments. The corrosion can lower the grain boundary resistance to cracking and even change the chemical makeup of the grain boundary zones. Stress cracking is the result of a triad of requirements: a susceptible alloy, high tensile stress, and a corrosive-specific environment.
Impingement Corrosion. This is caused by materials in a flow system, the flow system itself, and the flow system design. The direction of impingement appears to come from the opposite direction of the flow lines. The corrosion can be caused by particles in the flow mixture making contact with, and becoming embedded in, the piping system. It also can be caused by the flow of the mixture through turbulence, aeration, and wave erosion.
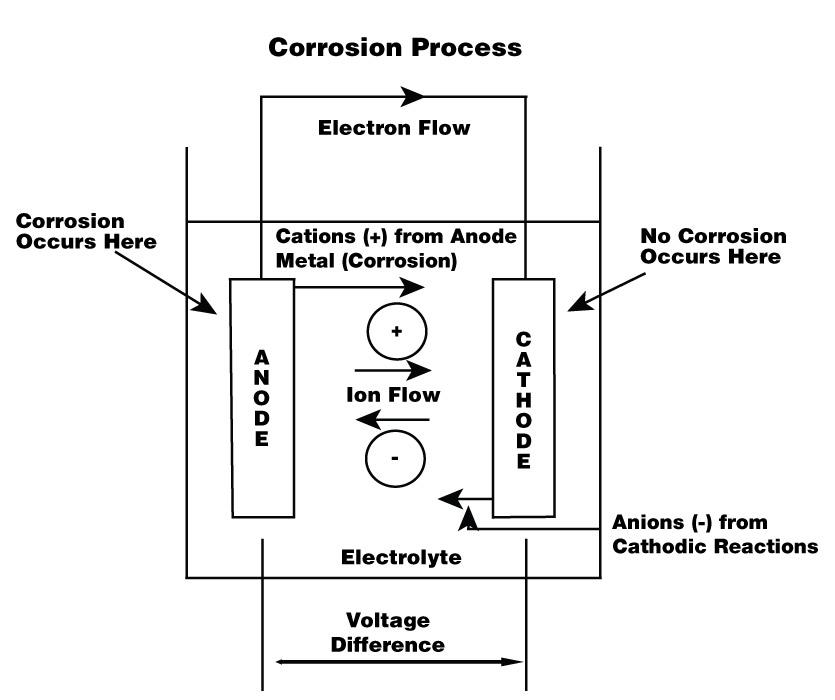
Figure 2
Corrosion occurs when anions and cations flow between an anode and a cathode, creating an electrical circuit. Note that the anode and cathode don’t need to have direct contact. They often are connected indirectly through a conducting medium, or electrolyte. In outdoor pipe installations, the conducting medium usually is soil; in indoor installations, it can be humid air.
The design of the piping system can contribute to the impingement and erosion of the inside walls of the pipes. Steep angles create impact zones and should be avoided in the design stage. Gentle angles and curves decrease impact likelihood and severity.
Micro-organism Corrosion. Anyone familiar with hydraulic system maintenance knows that bacteria can thrive in extreme environments, including high-temperature, high-pressure hydraulic circuits. Corrosion by such micro-organisms is one of the hardest forms of corrosion to stop. Micro-organisms can attack metal directly or indirectly. That is to say, some can actually ingest the metals, whereas others deposit corrosion-causing chemicals onto metals.
Stopping this type of attack usually involves chemicals that are benign to the metal but hostile to micro-organisms.
Mitigating Corrosion
Because corrosion develops in an electrical circuit, stopping corrosion is a matter of disrupting the electrical circuit.
Cathodic Protection. Cathodic protection (CP) is by far the best way to stop corrosion on pipelines. It uses impressed currents from a fixed anode to interfere with the electrical circuit in the corrosion cell. It is 100 percent effective against most forms of external pipe corrosion.
Galvanic CP connects a high-energy metal, such as zinc or magnesium, to the pipe (anode) (see Figure 4). The zinc or magnesium acts as a sacrificial anode, protecting the pipe. The sacrificial anode operates as a galvanic anode with impressed voltages as high as -1.4 to -2.1 V (and in some cases, even more negative).
If a counter-impressed current from a sacrificial anode is more negative than -0.85 V, corrosion in steel stops. Aluminum corrosion stops if the counter-impressed current is between -0.8 V and -1.00 V. If the current magnitude exceeds -1.00 V, an alkaline solution forms on the aluminum and attacks the metal. In some cases, a shift as small as -0.003 V is enough.
Proper CP levels also can be achieved with an impressed current system that uses a DC rectifier to drive the current into a suitable stable anode, such as carbon, platinized titanium, or magnetite (see Figure 5). The rectifier is connected at one end to the pipeline and at the other end to the impressing current anode, or bed of anodes. The rectifier current then is turned up until a reading of the pipeline voltage, relative to a copper sulfate half-cell, is less than -0.85 V. Care must be taken not to go to high, or coating disbondment will occur.
Electrical bonding. This method is used to stop stray AC and DC electrical current corrosion. It is a simple application of a conductor between the pipeline and the grounding system of the offending current source. The bonding wire provides a safe way back to the other utility’s grounding system that does not come from the surface of the pipeline. It also provides a safety element to protect workers from potentially fatal electrical shocks that can occur on insulated pipelines that run near high-voltage power lines and electric train and trolley systems.
Electrical bonding also can be achieved through the liberal use of galvanic anodes that place the pipeline at the same voltage potential as the offending utility.
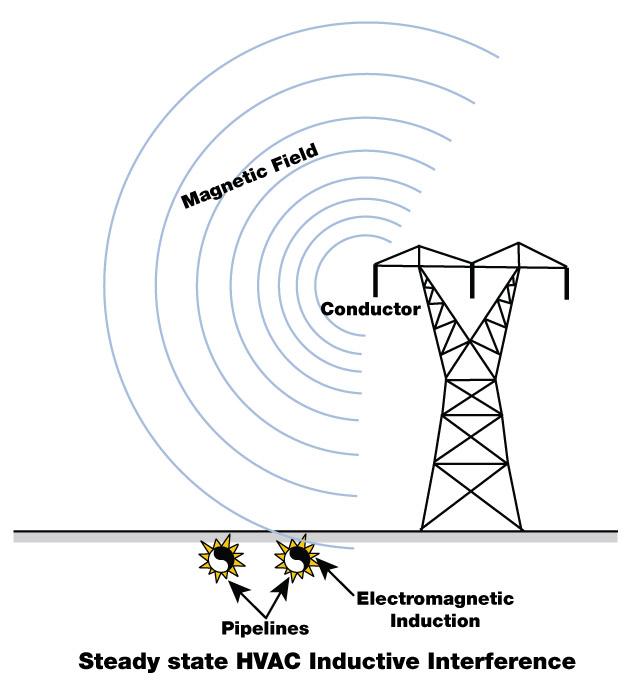
Figure 3
Induction creates a current in conductors near magnetic fields. This is the same as the induction welding process used in making much of the tube and pipe used in industry.
Care must be taken to be sure all pipe flanges are in electrical contact. If they are not in contact with each other, installing jumper wires from one to the next ensures that the entire pipeline is protected.
Coatings. Coatings can be used to stop or mitigate corrosion on both inside diameters (IDs) and outside diameters (ODs). Thousands of coating systems are available. To select the proper coating system for the job, the engineer needs to consider the pipe’s design, environment, contents, pressures, external influences, design life, and costs. Conventional external coatings such as mastic and Pritec®, and many newer types such as epoxies and polymers, have proven to be successful corrosion inhibitors. Internal coatings are available for contents corrosion control, cable pulling friction reduction, and erosion (impingement) corrosion control.
While coatings can fail in a variety of ways, adhesive failure, cohesive failure, and delamination are the main challenges.
The galvanic coatings used to stop corrosion include galvanization; aluminization; and GALVALUME®, which is a combination of aluminum and zinc. They usually are applied by hot dip, spray, or plasma spay. They work by providing CP to the surface. Their effectiveness is related to their thickness, damaged coating hole size, and atmosphere. Galvanization works well away from highly contaminated environments such as coastal zones, industrial pollution zones, and heavy agriculture pollution zones. In such locations, acids, salts, and other chemicals in the air readily attack the exposed anodic coatings. Also, impingement corrosion from flying sands pollutant mixtures (such as salt) quickly degrades the coating thicknesses and causes the coating systems to fail.
These systems can be quite successful if a top coating of a suitable material is properly installed before pipe installation system.
Inhibitors. Inhibitors generally are used to control corrosion on the inside of pipelines. The specific inhibitor depends on the material to be protected and the solutions transported inside the pipe.
For external protection, several types are available. Some absorb moisture so it can be collected in strainers. Others are developed to react with specific corrosion-causing agents to render them inert. Still another type is used to augment a concrete coating, greatly increasing the concrete’s protective capability.
Inhibitors are so numerous and have such a variety of capabilities that they can solve many problems that formerly seemed unsolvable.
About the Author
William A. Byrd, P.E., C.S.
803-781-7112
About the Publication
subscribe now
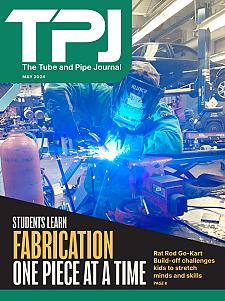
The Tube and Pipe Journal became the first magazine dedicated to serving the metal tube and pipe industry in 1990. Today, it remains the only North American publication devoted to this industry, and it has become the most trusted source of information for tube and pipe professionals.
start your free subscription- Stay connected from anywhere
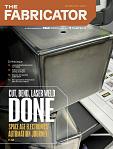
Easily access valuable industry resources now with full access to the digital edition of The Fabricator.
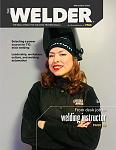
Easily access valuable industry resources now with full access to the digital edition of The Welder.
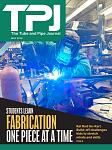
Easily access valuable industry resources now with full access to the digital edition of The Tube and Pipe Journal.
- Podcasting
- Podcast:
- The Fabricator Podcast
- Published:
- 04/16/2024
- Running Time:
- 63:29
In this episode of The Fabricator Podcast, Caleb Chamberlain, co-founder and CEO of OSH Cut, discusses his company’s...
- Trending Articles
Zekelman Industries to invest $120 million in Arkansas expansion
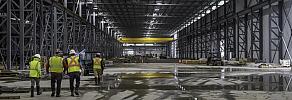
3D laser tube cutting system available in 3, 4, or 5 kW
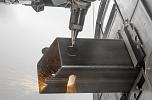
Corrosion-inhibiting coating can be peeled off after use
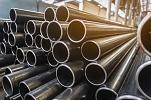
Brushless copper tubing cutter adjusts to ODs up to 2-1/8 in.
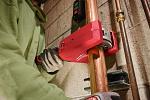
HGG Profiling Equipment names area sales manager
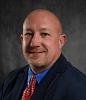
- Industry Events
16th Annual Safety Conference
- April 30 - May 1, 2024
- Elgin,
Pipe and Tube Conference
- May 21 - 22, 2024
- Omaha, NE
World-Class Roll Forming Workshop
- June 5 - 6, 2024
- Louisville, KY
Advanced Laser Application Workshop
- June 25 - 27, 2024
- Novi, MI