- FMA
- The Fabricator
- FABTECH
- Canadian Metalworking
Categories
- Additive Manufacturing
- Aluminum Welding
- Arc Welding
- Assembly and Joining
- Automation and Robotics
- Bending and Forming
- Consumables
- Cutting and Weld Prep
- Electric Vehicles
- En Español
- Finishing
- Hydroforming
- Laser Cutting
- Laser Welding
- Machining
- Manufacturing Software
- Materials Handling
- Metals/Materials
- Oxyfuel Cutting
- Plasma Cutting
- Power Tools
- Punching and Other Holemaking
- Roll Forming
- Safety
- Sawing
- Shearing
- Shop Management
- Testing and Measuring
- Tube and Pipe Fabrication
- Tube and Pipe Production
- Waterjet Cutting
Industry Directory
Webcasts
Podcasts
FAB 40
Advertise
Subscribe
Account Login
Search
Grain size, Part II: How metal grain size affects a bending operation
Better grade of material saves production time and cost on press brake
- By Steve Benson
- October 14, 2019
- Article
- Bending and Forming
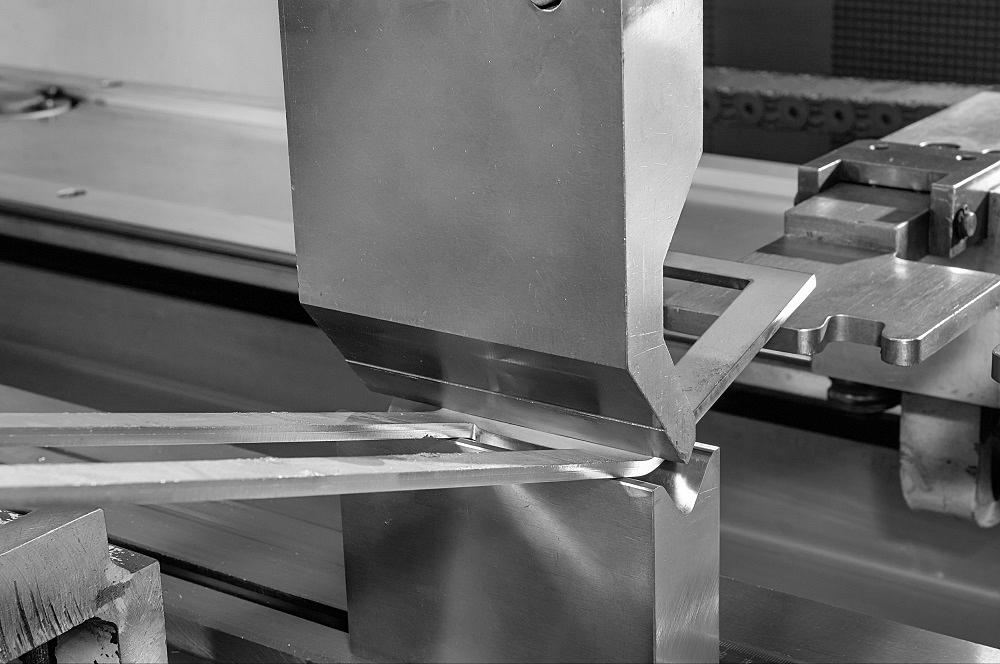
In the second part of this series, bending guru Steve Benson describes how material grain size affects a bending operation. Getty Images
Last month we dove into the weeds to understand material grains in sheet metal and plate, how they form, and what determines their size and orientation. This month we’ll dive into how exactly those grains affect how a material forms on the press brake.
We ended with a dichotomy: Large-grained materials are more ductile, small-grained materials are stronger and less ductile—and yet small-grained materials still can be easier to form on a press brake than certain large-grained materials. In fact, form very coarse-grain material, and you’ll find tearing and orange-peeling on the outside radius, especially if you’re making a sharp bend. What gives? To find out, read on.
A Quick Review
Steel and other metals are made up of very small groupings of molecules, and they’re arranged so that they generally resemble a cube. The length of these groupings is smaller than one-tenth of a millionth of an inch on each side. While you will be unable to see these groupings of molecules, we can see the effects of their presence. These groupings of molecules manifest as irregularly shaped crystals or crystal-grains.
Because of the extremely large number of molecules that make up any material, these crystal-grains become visible to the naked eye. When the metal has been cold-rolled or cold-worked, the crystals elongate and take a directional preference in the direction of roll, creating the grains we see.
The size and number of grains a material has is guided by the rate of the metal’s solidification from its liquid state. Steelmaking starts with molten metal that solidifies as the material is cooled. As it cools, recrystallization occurs, at which point higher-temperature, new, strain-free grains nucleate and grow inside the old distorted grains and at the grain boundaries. With recrystallization, the material’s mechanical properties return to their original, weaker state, becoming much more ductile.
A crystalline material’s ability to plastically deform depends on its capacity for dislocation; that is, the grains’ ability to move freely within a material. Grain boundaries—areas with a surplus of molecules that are not part of any symmetrical crystal formation—act as a barrier to that dislocation, where the crystals do not slip easily over one another. A decreasing grain size increases the number of borders (grain boundaries) that must give way before movement can occur. The smaller the grain size, the stronger the material.
Slowing the movement of grain dislocations also strengthens the material. And there are any number of ways to reduce the dislocation movement. These include alloying and strain hardening.
Control of Grain Size
Larger grains reduce the strength and toughness of the material, and grains can grow for various reasons. For example, if the material is left at above recrystallization temperature for too long, the grains increase in size as diffusion occurs across the grain boundaries.
A material’s grain size affects the material’s strength because, again, the grain boundary acts as a barrier to dislocation, resulting in movement along a slip plane. This is because adjoining grains have different orientations (see Figure 1). In material with small grains, the distance that a particle can move along the slip plane is shorter. This reduced movement among smaller grains increases the material’s strength.
Grain Direction and the Bend Line
The sheet or plate material is the weak link in forming. We should always consider as many material variables as possible before we commit a project for production. And grain size is one of the major variables. Ideally, the material grain needs to be considered in depth before the design phase is finished and again before the purchase order is released—that is, if you want to reduce the number of problems that will manifest during production.
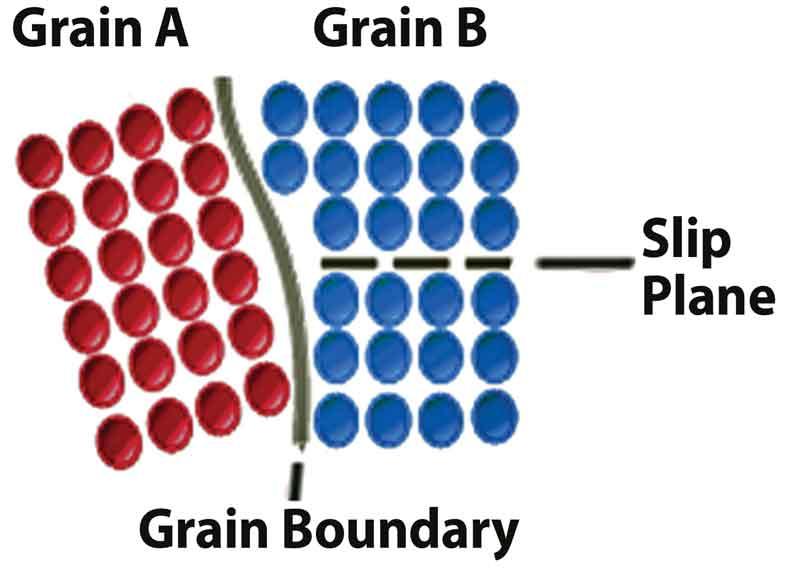
Figure 1
A grain boundary affects how a material’s particles can move along the slip plane, acting as a barrier to dislocation.
If you have cracking or orange-peeling on the outside surface of bends, material grain orientation might be the problem. At the press brake, it’s always best practice to place your bend lines so they are perpendicular to the material’s grain direction. Of course, that’s not always practical or possible, especially in parts containing multiple bends that are both with and against the grain. If having bending lines perpendicular to the grain isn’t practical, try bending diagonal to the grain.
Annealing and Normalizing
When a strain-hardened material is exposed to elevated temperatures, the strengthening that results from the plastic deformation of forming can be lost—a bad situation if the metal needs that strength to support some load. Nonetheless, the strengthening caused by strain hardening is not always desired, especially if you need higher ductility to make multiple bends. Heat treatments can remove the strain-hardening effects.
Regardless of the grain size produced at the mill, you can manipulate the grain size in fabrication, even after forming at the brake. The material’s crystals can be made to become more uniform using heat-treating processes like annealing and normalizing.
Normalizing is a process in which the material is heated to just below the point of recrystallization and then allowed to cool in the open air. Annealing is done by reheating the material to just below the point of recrystallization, but rather than air cooling, it is brought back to room temperature slowly without removing the material from the furnace. Of the two different methods, normalizing produces the finest grain structure.
Three events occur during heat treating: recovery, when the grains recover slightly from cold working; recrystallization, when new grains form; and, finally, grain growth, when larger grains grow at the expense of smaller grains. As shown in Figure 2, hardness and strength drop during recrystallization, and as the grains grow, so does the material’s ductility.
Held at an elevated temperature, a strain-hardened material can relieve some internal strain energy. Molecules are not in fixed locations and can move around when enough energy is supplied to break the bonds that are holding them in place. Rising temperatures rapidly increase the amount of diffusion. This allows those molecules that are in extremely strained locations to move to areas that are less strained.
This is the recovery phase that allows for an adjustment in the strain on a minuscule scale. It changes the dislocation density and shifts locations to a lower energy state, reducing internal residual stress in the workpiece.
Specifying Grain Size
ASTM International specifies grain size numbers that can be used to determine the number of grains per square inch at 100x magnification (see Figure 3). The higher the grain-size number, the smaller the average grain size. HSLA steels often have grain-size numbers ranging from 10 to 12. Traditional low-strength forming steels have grain-size numbers around 6 or 7. Grain-size numbers of 5 and lower can have visual surface problems like cracks, tears, and orange peels.
Remember that the grain boundaries are stronger than the grain interior. When the steel is stretched to large strain levels, the grain boundaries resist deformation and allow the core of the grain to deform. This obviously is not acceptable for a Class A finish, making it advisable to specify a grain-size number of 6 or higher.
Below 1, ASTM specifies grain sizes of 0 and 00, both of which have less than 1 grain per square inch at 100x magnification. Annealing can sometimes make a material’s grains grow to 00 or even larger. Any bends placed in that material will be susceptible to tears or cracks on the outside of the bend radius. The outside surface might resemble an orange peel or have small divots. If you have encountered one of these issues, grain size is the culprit, along with a too small inside bend radius. The deeper you go into the sharp-bending realm, the worse the effect becomes.
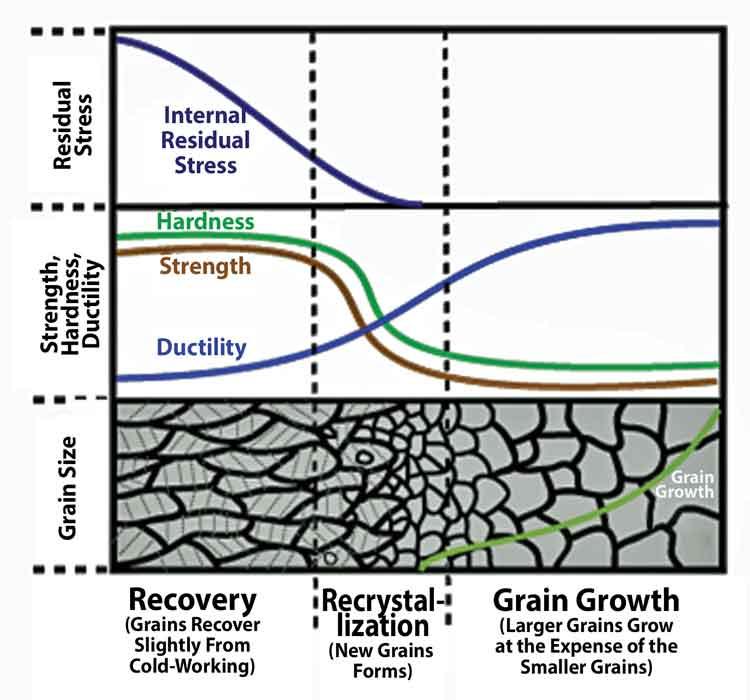
Figure 2
Recovery, recrystallization, and grain growth relate to grain size, hardness, ductility, and residual stress in the material.
Ductility, Grain Size, and Formability
The mechanical properties of sheet or plate change with a reduction in grain size. Compared to fine-grained materials, coarse-grained materials are not as hard, have a lower yield strength, and are more ductile. Ductile material forms better with less cracking, tearing, or orange-peeling. Though as described previously, excessive large grains can create problems too.
A finer grain size means more grain boundaries, and more grain boundaries means a greater resistance to dislocation. It is the measured ability of a material to withstand serious plastic deformation, making the material less ductile.
But wait, how can material with a finer grain size be less ductile and yet withstand more plastic deformation without failure at the same time? For the most part, it comes down to probability. The higher the grain count, the more likely it is that some of them will be oriented in such a way that the strain is being applied to slip planes. The more grains you have, the more slip planes are oriented in a similar direction, and, therefore, there will be a greater amount of deformation without failure, like cracking, splitting, or orange-peeling. (As a side note, this is the essence of anisotropy and isotropy; for more on this, see Material grain considerations for press brake operations.)
Of course, it is not as simple as probability alone; other factors are in play. If the grain or crystals are small enough, the dislocation motion ceases to be the main mode of plastic deformation. Other components of plastic behavior come into play, including grain boundary sliding, in which grains move relative to one another. Grain boundary sliding can occur in larger-grained material, but on a limited basis. Flow stress, or the stress required to sustain plastic deformation at a given level of strain, also comes into play.
Another driver of plastic behavior is shear localization. If prone to shear localization, a material will experience a strengthening of the grain boundaries. The orientation of grains can suppress grain boundary sliding, allowing the more ductile metal to deform more easily. Again, this is a function of anisotropy and isotropy and is of great importance to the person operating the press brake.
A finer grain size means a greater density of grain boundaries, which affects a material’s ductility in different ways. Grain boundaries are known for dislocation-anchoring, which lowers ductility. Finer grains also mean there are more grain boundaries. The greater the number of grain boundaries, the greater the tonnage is required to bend the metal. This happens because the energy required to produce movement at the boundaries is stronger than the grain itself.
At the same time, a grain boundary that is finer is known to raise ductility. When the density of grain boundaries increases, these dislocations are anchored and evenly distributed within the material.
Grain Size and Springback
Springback also changes with grain size. In previously published research, coarser-grained material required minimal if any springback compensation, while fine-grained materials showed a large amount of springback that needed to be compensated for, be it through real-time angle monitoring or through the tool selection process.
The Value of Good Material
All this new understanding of crystallization, grain, and grain direction boil down to this: By purchasing a better grade of material, you could save a great number of hours of production time and cost.
Of course, while some customers will not allow you to upgrade the material for mechanical and design reasons, most are not going to complain, especially if you’re not charging them for the upgrade. True, your materials cost will go up a small amount, but you will make up for that and more just in labor savings.
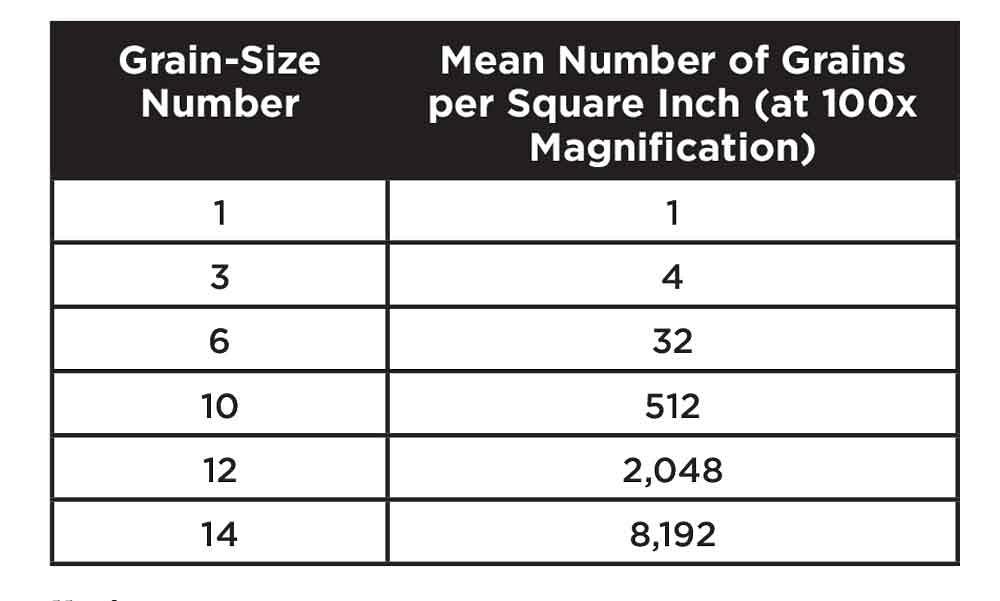
Figure 3
ASTM International designates grain-size numbers on the basis of the average number of grains a material has within a specified area.
As a bonus, you’ll improve product quality, which will improve shop morale, make customers happier, and could lead to a growing customer base, even if it’s only by word-of-mouth. Why? Because everyone loves good quality at a fair price.
Steve Benson is a member and former chair of the Precision Sheet Metal Technology Council of the Fabricators & Manufacturers Association International®. He is the president of ASMA LLC, steve@theartofpressbrake.com. Benson also conducts FMA’s Precision Press Brake Certificate Program, which is held at locations across the country. For more information, visit fmanet.org/training, or call 888-394-4362. The author’s latest book, Bending Basics, is now available at the FMA bookstore, fmanet.org/store.
About the Author
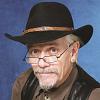
Steve Benson
2952 Doaks Ferry Road N.W.
Salem, OR 97301-4468
503-399-7514
Related Companies
subscribe now
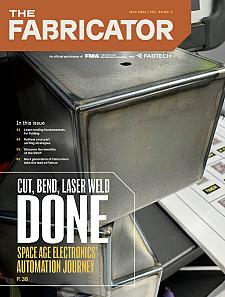
The Fabricator is North America's leading magazine for the metal forming and fabricating industry. The magazine delivers the news, technical articles, and case histories that enable fabricators to do their jobs more efficiently. The Fabricator has served the industry since 1970.
start your free subscription- Stay connected from anywhere
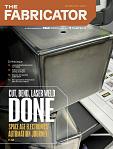
Easily access valuable industry resources now with full access to the digital edition of The Fabricator.
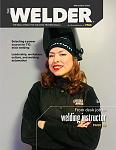
Easily access valuable industry resources now with full access to the digital edition of The Welder.
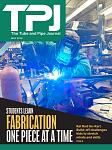
Easily access valuable industry resources now with full access to the digital edition of The Tube and Pipe Journal.
- Podcasting
- Podcast:
- The Fabricator Podcast
- Published:
- 04/16/2024
- Running Time:
- 63:29
In this episode of The Fabricator Podcast, Caleb Chamberlain, co-founder and CEO of OSH Cut, discusses his company’s...
- Trending Articles
Capturing, recording equipment inspection data for FMEA
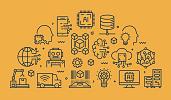
Tips for creating sheet metal tubes with perforations
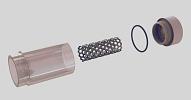
Are two heads better than one in fiber laser cutting?
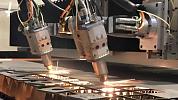
Supporting the metal fabricating industry through FMA
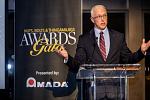
Omco Solar opens second Alabama manufacturing facility
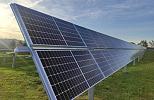
- Industry Events
16th Annual Safety Conference
- April 30 - May 1, 2024
- Elgin,
Pipe and Tube Conference
- May 21 - 22, 2024
- Omaha, NE
World-Class Roll Forming Workshop
- June 5 - 6, 2024
- Louisville, KY
Advanced Laser Application Workshop
- June 25 - 27, 2024
- Novi, MI