Graduate Research Associate
- FMA
- The Fabricator
- FABTECH
- Canadian Metalworking
Categories
- Additive Manufacturing
- Aluminum Welding
- Arc Welding
- Assembly and Joining
- Automation and Robotics
- Bending and Forming
- Consumables
- Cutting and Weld Prep
- Electric Vehicles
- En Español
- Finishing
- Hydroforming
- Laser Cutting
- Laser Welding
- Machining
- Manufacturing Software
- Materials Handling
- Metals/Materials
- Oxyfuel Cutting
- Plasma Cutting
- Power Tools
- Punching and Other Holemaking
- Roll Forming
- Safety
- Sawing
- Shearing
- Shop Management
- Testing and Measuring
- Tube and Pipe Fabrication
- Tube and Pipe Production
- Waterjet Cutting
Industry Directory
Webcasts
Podcasts
FAB 40
Advertise
Subscribe
Account Login
Search
R&D Update: Predicting springback when bending AHSS and aluminum alloys, Part II
Inverse analysis results and case studies
- By Aanandita Katre, Ali Fallahiarezoodar, and Taylan Altan, Ph.D.
- January 12, 2017
- Article
- Bending and Forming
Nonlinear Unloading Behavior of Materials
When finite element (FE) simulation is used for predicting metal flow and springback, the material elasticity typically is defined by the handbook value of the Young’s (E) modulus. The common way to determine the E-modulus is through a tensile test. However, the unloading behavior of the material usually is nonlinear because of unloading strains resulting from the repelling dislocations moving away from each other in addition to atomic bond relaxation when the applied stress is reduced.
Since the unloading behavior of metals following the plastic deformation is nonlinear, defining the material response using a constant E-modulus is not correct, and the resulting prediction of springback is inaccurate.
Material Models for Accurate Springback Prediction
Several methods and constitutive models have been developed to improve springback prediction that consider the isotropic/anisotropic and kinematic hardening behavior of materials; the effect of friction; and the complex behavior of material, such as Bauschinger effect, transient hardening, and permanent softening.
Some of these models, such as the Yoshida-Uemori model, the quasi-plastic-elastic model, and the homogeneous anisotropic hardening model, have shown some improvement in springback prediction.
However, in most cases, several specific parameters have to be determined through different testing processes, such as the loading/unloading tensile test or the tension compression test, before the model can be used. The need for these extensive testing processes limits the industrial application of these models, especially considering batch-to-batch variations of material properties for the most currently available advanced high-strength steels (AHSS).
Furthermore, in most of these models, the required coefficients are developed using a uniaxial tension/compression test at relatively low strain. In actual stamping, however, the deformation can be in a different strain state (plane strain or biaxial), and strains can be larger.
Inverse Analysis
Research is ongoing at the Center for Precision Forming (CPF) at The Ohio State University to develop a practical method, called the inverse analysis method, to increase the accuracy of springback prediction. The objective is to reduce the prediction error and consequently save the cost and time for die modification and recut for springback compensation.
Flow stress and the E-modulus are the two most important material parameters for predicting springback. Therefore, accurate determination of E-modulus can improve springback prediction.
In the inverse analysis method, a constant apparent E-modulus can be used to predict springback determined via a bending test and FE simulation of the bending process. In this study, FE simulations were run in PAM-STAMP® using shell elements. The FE simulation was performed initially using the constant handbook value of the E-modulus, which usually is determined by the tensile test. A second simulation then was performed using another E-modulus value. The springback predicted by the second simulation was again compared with the experimental result. Finally, the appropriate apparent E-modulus for accurate prediction of springback was estimated (see Figure 1).
Inverse analysis has been tested in the following situations:
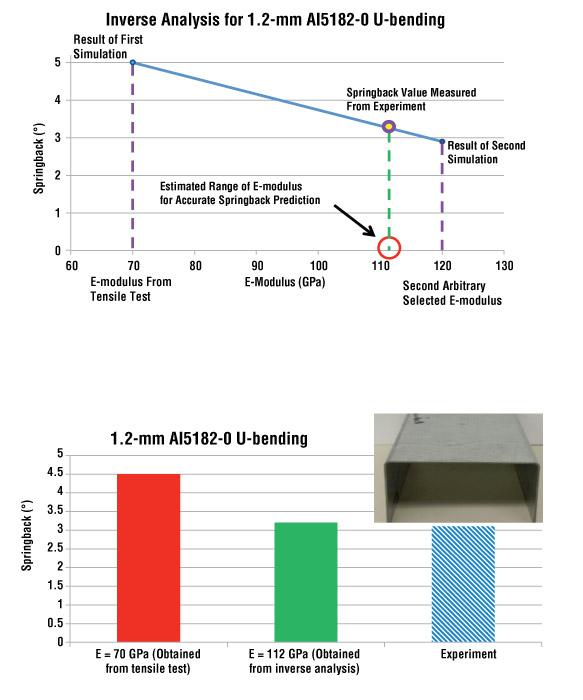
Figure 1
The appropriate average E-modulus for accurate springback prediction is determined by comparing the results of two simulations and experimental data.
- U-bending of several different AHSS and aluminum alloys. Results show a significant improvement of springback prediction (see Figure 2).
- An industrial part made from DP980 material (see Figure 3). The part geometry and the experimental data were provided by Die Cad Group. Figure 4 shows the predicted results compared to the 3-D scanned data of the panel formed in the tryout. The contour colors indicate the normal distance, in millimeters, of the predicted part geometry after springback to the actual experimental results. As shown, when the E = 120 GPa was used in the simulation, the springback prediction was closer to the real part than the simulation result with the handbook value of the E-modulus for DP980 (E = 205 GPa).
Limitations of the Inverse Analysis Method
The inverse analysis method has two main limitations:
- The elastic recovery of the material is a function of plastic strain. Therefore, the E-modulus as a parameter that determines the elastic recovery should be different for different strain values and distribution. The E-modulus determined through inverse analysis for a specific bending process is valid only for that bending operation and material thickness.
- The real bending test with the actual tooling should be conducted to determine the apparent E-modulus. Therefore, this method can only reduce the number of die modifications and recuts during die tryout.
References
J.Y. Lee, J.W. Lee, M.G. Lee, F. Barlat, “An application of homogeneous anisotropic hardening to springback prediction in pre-strained U-draw/bending,
”International Journal of Solids and Structures, Vol. 49 (2012), pp. 3562–3572.Li Sun and R.H. Wagoner, “Complex unloading behavior: Nature of the deformation and its consistent constitutive representation,”
International Journal of Plasticity, Vol. 27 (2011), pp. 1126–1144.F. Yoshida et al., “Elastic-plastic behavior of steel sheets under in-plane cyclic tension-compression at large strain,”
International Journal of Plasticity, Vol. 18 (2002), pp. 633-659.About the Authors
Aanandita Katre
1971 Neil Ave. Room 339 Baker Systems Engineering Building
Columbus, OH 43210
Ali Fallahiarezoodar
Graduate Research Associate - Center for Precision Forming
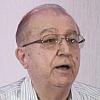
Taylan Altan, Ph.D.
Professor Emeritus and Director - Center for Precision Forming
subscribe now
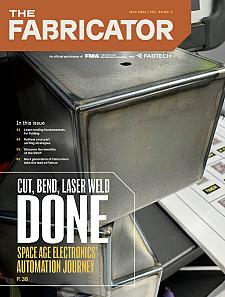
The Fabricator is North America's leading magazine for the metal forming and fabricating industry. The magazine delivers the news, technical articles, and case histories that enable fabricators to do their jobs more efficiently. The Fabricator has served the industry since 1970.
start your free subscription- Stay connected from anywhere
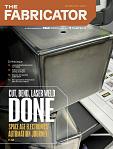
Easily access valuable industry resources now with full access to the digital edition of The Fabricator.
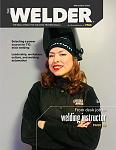
Easily access valuable industry resources now with full access to the digital edition of The Welder.
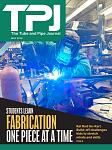
Easily access valuable industry resources now with full access to the digital edition of The Tube and Pipe Journal.
- Podcasting
- Podcast:
- The Fabricator Podcast
- Published:
- 04/16/2024
- Running Time:
- 63:29
In this episode of The Fabricator Podcast, Caleb Chamberlain, co-founder and CEO of OSH Cut, discusses his company’s...
- Trending Articles
Capturing, recording equipment inspection data for FMEA
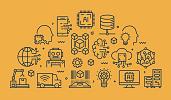
Tips for creating sheet metal tubes with perforations
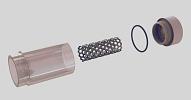
Are two heads better than one in fiber laser cutting?
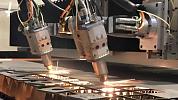
Supporting the metal fabricating industry through FMA
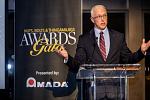
Omco Solar opens second Alabama manufacturing facility
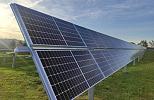
- Industry Events
16th Annual Safety Conference
- April 30 - May 1, 2024
- Elgin,
Pipe and Tube Conference
- May 21 - 22, 2024
- Omaha, NE
World-Class Roll Forming Workshop
- June 5 - 6, 2024
- Louisville, KY
Advanced Laser Application Workshop
- June 25 - 27, 2024
- Novi, MI